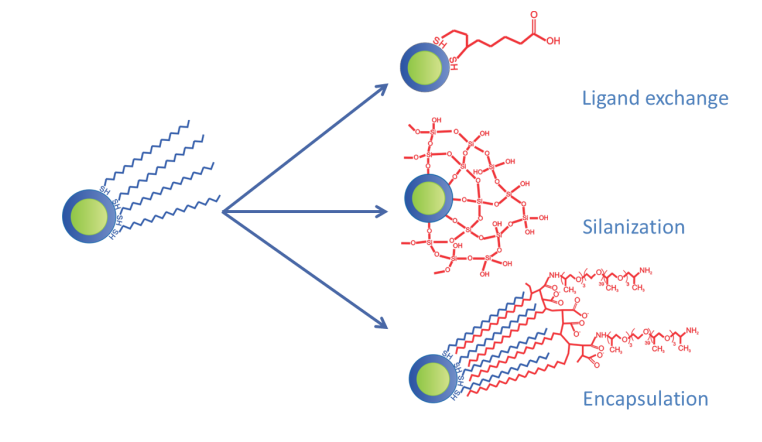
Bioconjugation reactions are most commonly performed in aqueous solutions rather than in organic solutions. Since bioconjugation uses biomolecules that function most effectively in conditions that thermodynamically mirror those in vivo, it is useful, if not vital, to use an aqueous solution. This not only allows properties like pharmacokinetics to be properly analyzed but also ensures that proteins and other biomolecules will fold and orient themselves in their native conformations so that selectivity can be activated for conjugation reactions. In this article we’ll discuss bioconjugation in water including protocols, challenges, and applications.
For bioconjugation in water, researchers utilize several chemistries including EDC and NHS chemistry, click chemistry, and aldehyde-hydrazine conjugation chemistry.
Challenges of Bioconjugation in Water
Challenges of bioconjugation in water include decreased efficacy of hydrophobic crosslinkers, hydrolysis of reagents, and quantum dot chemistries.
Challenge 1. Hydrophobic Linkers Can Lead to Aggregation in Water
Linkers and other reagents used in bioconjugation reactions can be hydrophilic or hydrophobic. Hydrophilic reagents exhibit increased solubility, stability, and specificity during bioconjugation in water. However, hydrophobic reagents, like maleimides and NHS-esters, which are frequently utilized, tend to have challenges in water. FITC conjugation, for example, needs to first be dissolved in DMSO and then in water because it is so hydrophobic.
There are a variety of reasons why hydrophobic linkers like maleimides and NHS-esters are utilized. They tend to be commercially available and easily functionalized with any necessary functional groups. Their reactions are also often well-characterized, therefore their limitations can be easily overcome.
One specific problem with hydrophobic linkers can be found in protein-protein bioconjugation. After using hydrophobic linkers for the reaction, hydrophobic elements are exposed and only attached to one protein. This increases the hydrophobicity of the conjugate and can lead to aggregation and precipitation of the proteins through hydrophobic interactions.
Increased hydrophobicity is also a concern for antibodies, as the drugs used in antibody-drug conjugates are often hydrophobic and therefore reduce the solubility of the ADC, which presents problems for in vivo active transport mechanisms.
There are also some applications in which these properties may be less of a detriment. For example, protein aggregation may help with fluorescent signal amplification and increased hydrophobicity of antibodies may allow them to more easily cross phospholipid bilayers.
Trying to attach molecules together? You can explore conjugation kits to help you attach biomolecules together quickly and repeatably here.
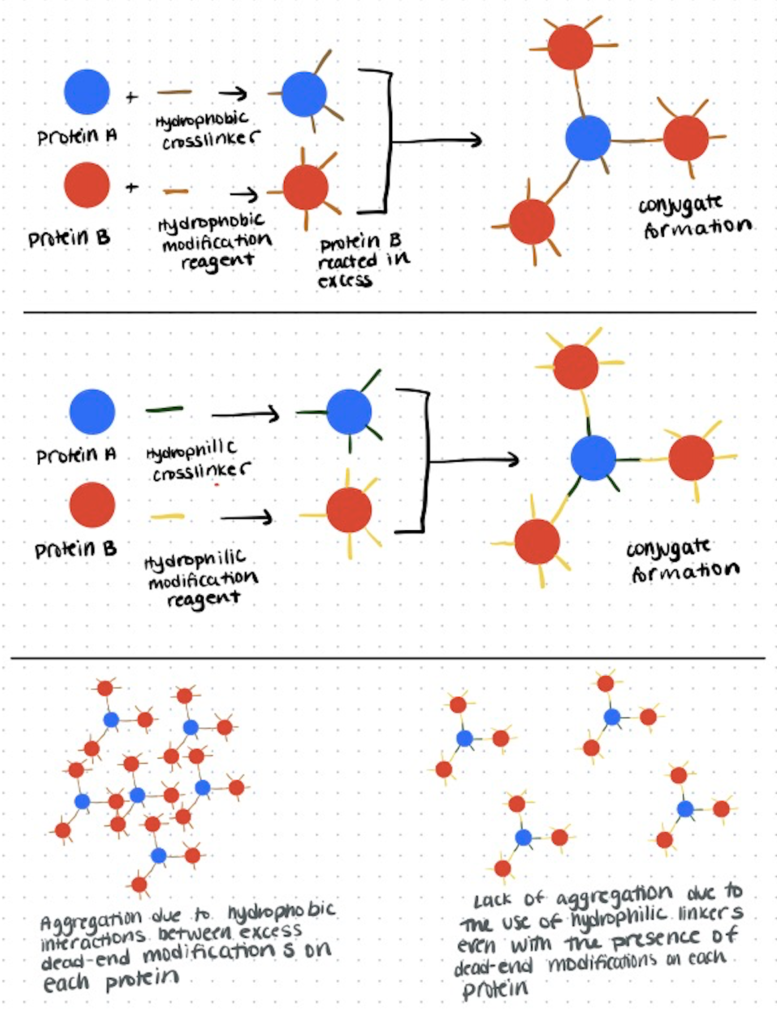
There are different methods that help to mitigate decreased efficacy from hydrophobic interactions. Small hydrophilic molecules such as polyethylene glycol can be conjugated to targets to restore some solubility and stability. Additionally, you can modify reaction conditions such as the pH and temperature so that conjugates are more stable. At times, an organic solvent like DMSO or acetonitrile may even be used to help solubilize reagents. You can also use rarer amino acids like tyrosines for bioconjugation reactions so you don’t have an overabundance of hydrophobic linkers.
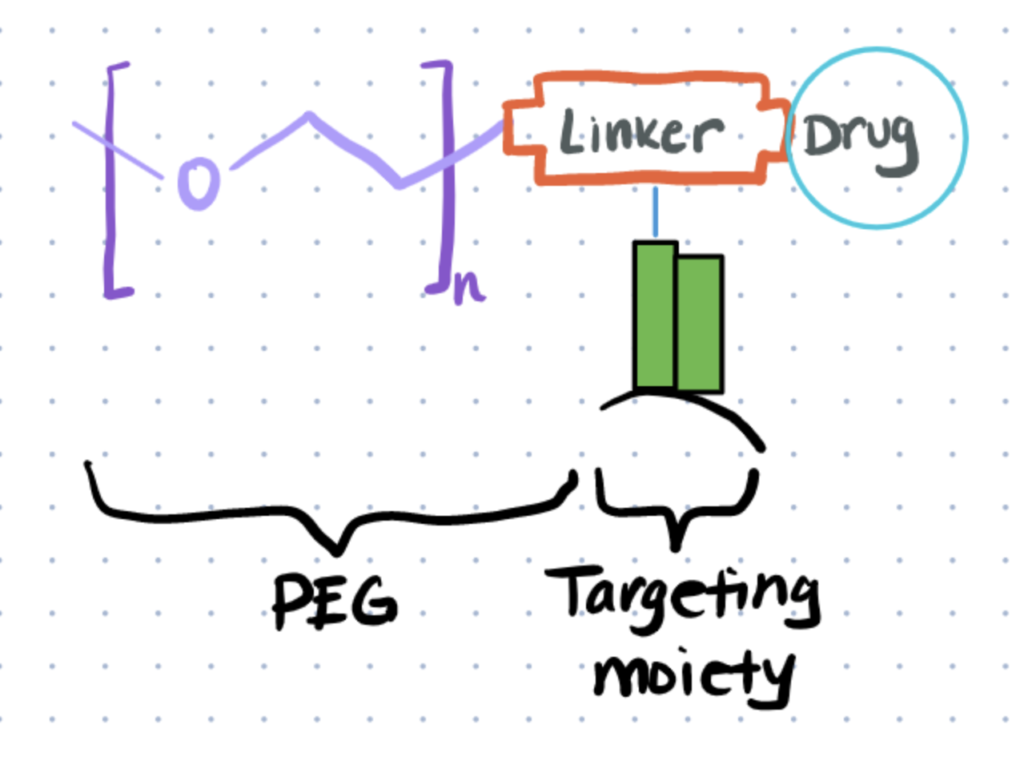
Challenge 2. Hydrolysis of Reactants
In an aqueous solution, some reagents like maleimides, NHS esters, and oximes can undergo hydrolysis. If left uncontrolled, this results in decreased yield of the conjugated product. The hydrolysis reaction can be pH-dependent, so adjusting the pH of the reaction buffer can help to minimize this effect. For example, hydrolysis of maleimides becomes more likely as the pH increases.
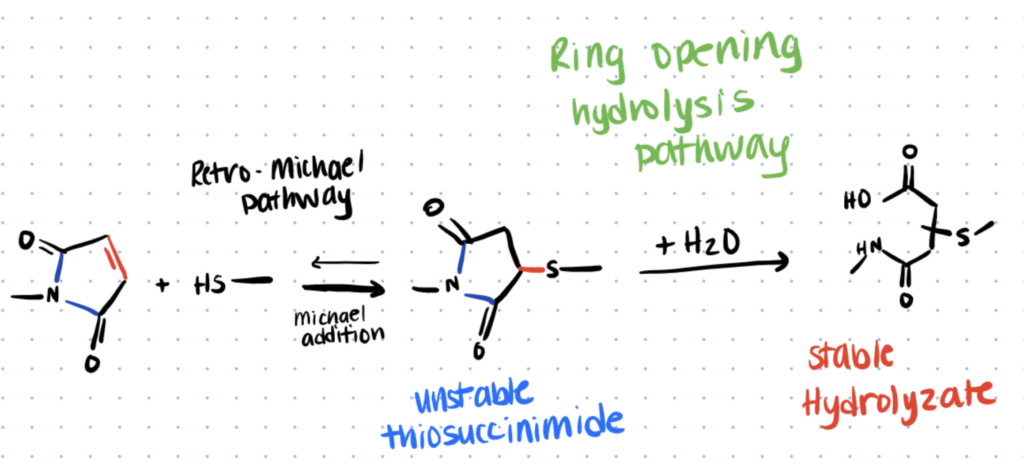
Challenge 3. Materials Like Quantum Dots Need Hydrophilic Shells for Bioconjugation in Water
Quantum dots (QDs) are semiconducting nanocrystals that have unique fluorophore characteristics. In many ways, they are replacing traditional fluorophores, as they have broader absorption spectra but narrow emission spectra, they have larger surface areas for functionalization, and they are more chemically stable. We have discussed bioconjugation of quantum dots in more detail in another article.
However, quantum dots are also inherently hydrophobic and must be hydrophilized. There are a few different strategies that can achieve this. The hydrophobic coating can be replaced with one that’s more hydrophilic on the exterior and can include functionalizing groups like thiols and amines. Alternatively, quantum dots can be coated with silica shells, which provide stability and can later be functionalized. Quantum dots can also be encapsulated in carrier vesicles, such as liposomes or amphiphilic polymers.
Related articles:
- Interested in conjugating quantum dots via thiols or arginines? You can read about bioconjugation of maleimides here and arginine bioconjugation as well. Maleimides are a classic strategy for reacting with thiols and arginines provide more site-selectivity since they are rarer.
- We also discuss other thiol-mediated bioconjugation techniques in a related article.
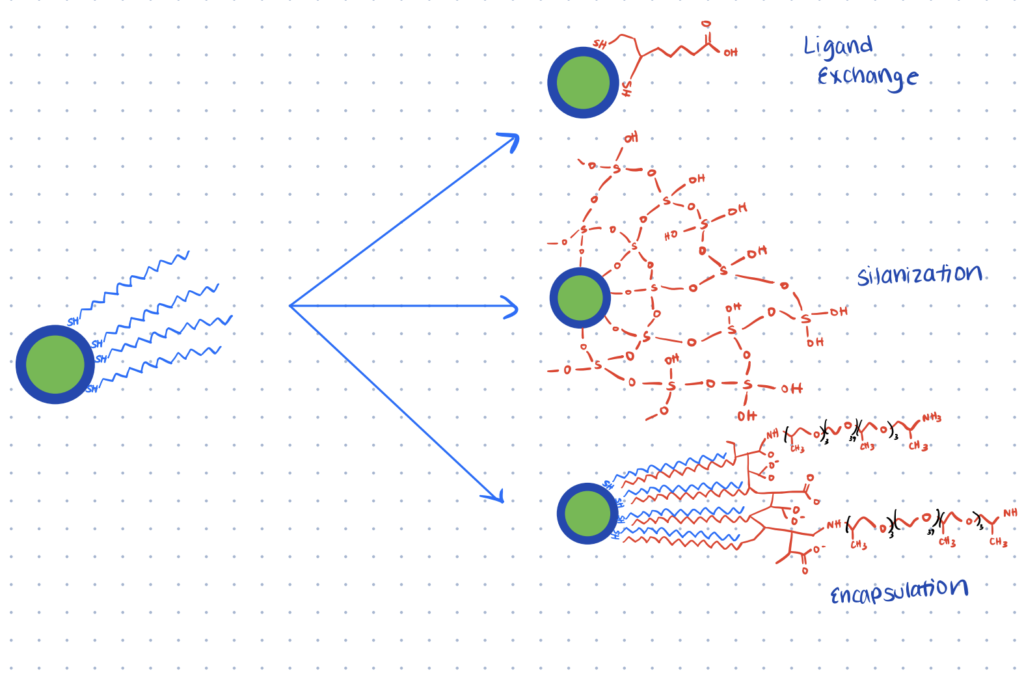
Separating Conjugates from Reagents During Characterization of Water-based Reactions
Dialysis, chromatography, and centrifugation can be used to separate products from unreacted reagents in water-based reactions.
Often during bioconjugation reactions in water, excess reagents will remain in the aqueous solution. It is important to separate the conjugated products from any unreacted excess so that they don’t interfere with assays, pharmacokinetics, or any other downstream applications. It’s also necessary to remove excess reagents when using a technique like LC-MS (liquid chromatography-mass spectrometry) to determine if the bioconjugation reaction was successful.
One method for removal is dialysis. This process utilizes semipermeable membranes and concentration gradients to separate molecules based on their size.
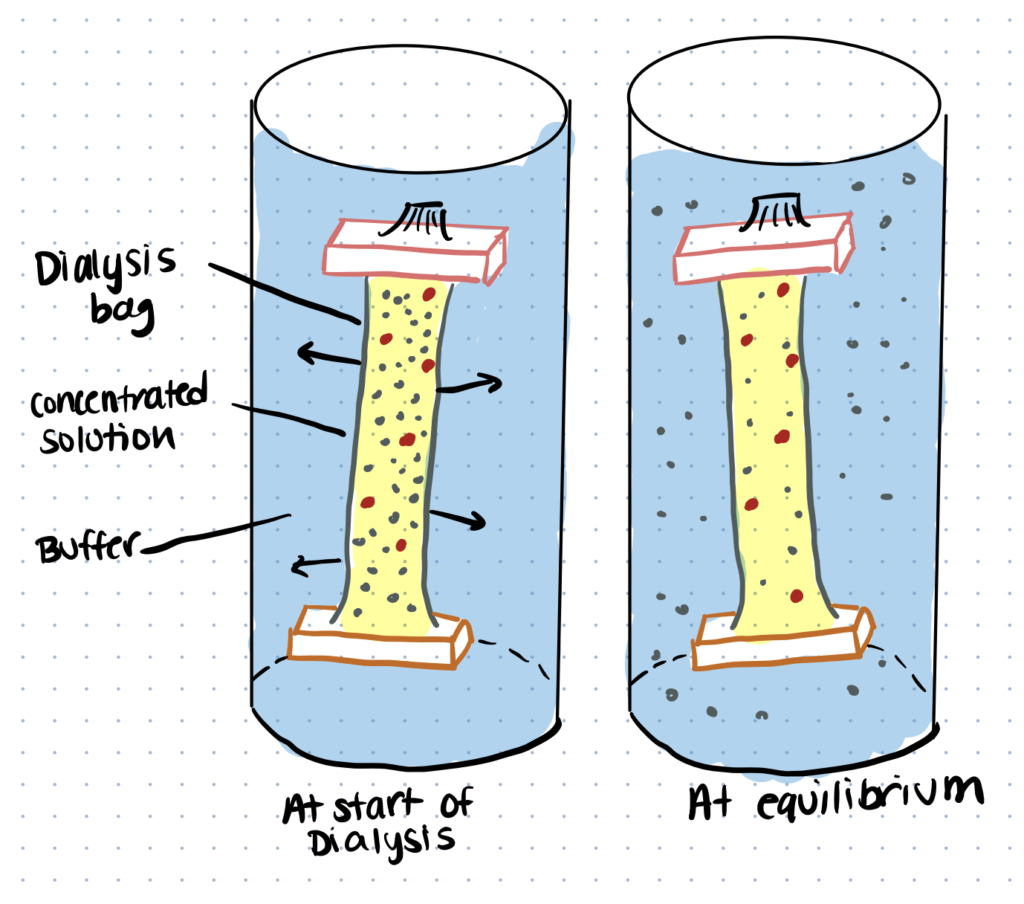
Chromatography is another common technique to purify bioconjugates. As you pass media through a chromatography column, certain molecules will be captured and separated from the rest. There are many different kinds of chromatography — particles can be separated by attributes like size or charge. Therefore, this method can be customized depending upon what properties distinguish the conjugated vs. unconjugated reagents.
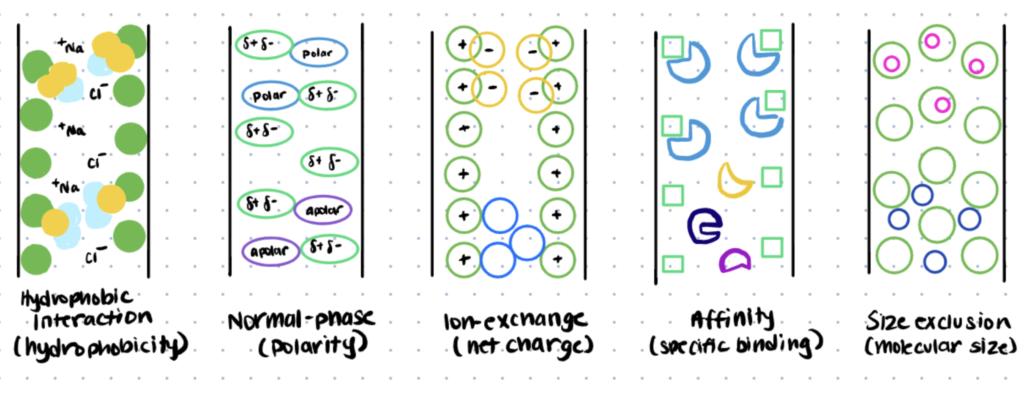
Centrifugation can also be used to separate out conjugates from starting materials. Here, larger and denser particles will sediment more quickly when subjected to high speeds. These pellets can be collected and resuspended in new media, free from other reagents. If you use techniques such as electrochemical bioconjugation then you won’t need to separate as many conjugation reagents since solid electrodes are frequently used.
A Method for Bioconjugation of Peptides In Water
Peptide bioconjugation is used widely in biomedical research. Totaro et. al (2016) investigated peptide bioconjugation in aqueous media using EDC/sNHS (1-Ethyl-3-(3-(dimethylamino)propyl)carbodiimide/ N-hydroxysulfosuccinimide) as a conjugation agent. Read this article for their complete protocol.
Most proteins and antibodies have readily available amines and carboxyls. You can label proteins and antibodies with these conjugation kits to save time and improve consistency between experiments.
Step 1. Synthesize Peptides
Synthesize peptides with the sequence Ac-Xaa-Leu-Gly-Glu-Gly-Leu-CONH2 using Fmoc-SPPS (fluorenylmethoxycarbonyl-solid-phase peptide synthesis) chemistry.
Step 2. Conjugate 2-(2-pyridyl)ethylamine to Peptides
Mix sNHS with 2-(2-pyridyl)ethylamine and add to a peptide stock solution in a reaction buffer comprised of 1 M MOPS (3-(N-morpholino)propanesulfonic acid) and 1M NaCl at a pH of 7.1. Add the EDC stock solution. Vortex and leave at room temperature for 20 hours, then analyze with LC-MS (liquid chromatography–mass spectrometry).
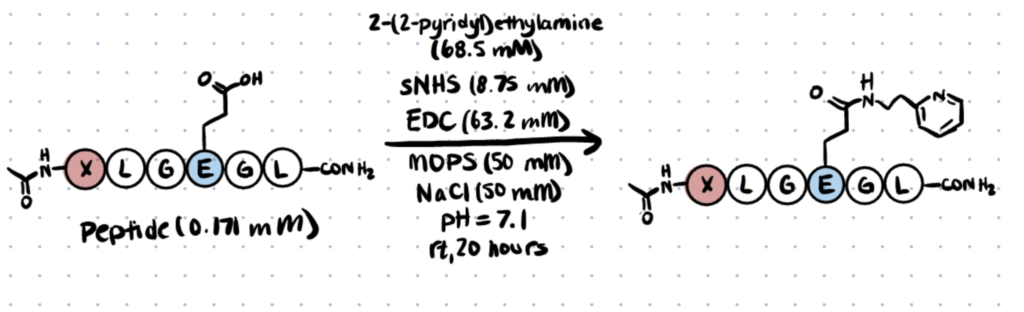
Oligonucleotide Bioconjugation in Water
Antibody-oligonucleotide conjugates can be used as an alternative to antibody-drug conjugates, as well as in protein diagnostics. Here we present a method from Wiener et. al (2020), in which they created soluble antibody-oligonucleotide conjugates using the click reaction. Read this article for more expanded methods.
Step 1. Conjugate the Antibody with DBCO Crosslinker
Adjust concentrations of anti-Igfr-L1 antibodies to 1 mg/ml in PBS (phosphate-buffered saline). Dissolve DBCO-NHS (dibenzocyclooctyne-N-hydroxysuccinimide) in anhydrous DMSO (dimethyl sulfoxide), adjust to 10 mM, then mix with the antibody solution for 1 hour. Remove unreacted reagents with dialysis.
Step 2. Functionalize the Oligonucleotide with Azide Groups
Dissolve 3AA-NHS (3-azidopropionic acid sulfo-NHS ester) in anhydrous DMSO at 10 mM. Then, dissolve amine-functionalized oligonucleotides in PBS at 100 µM. Add the first solution to the second until 10 times molar excess of 3AA-NHS is reached, then incubate at room temperature for 2 hours and remove excess reagent with dialysis.
Step 3. Conjugate the Oligonucleotide with the Antibody
Add azide-functionalized oligonucleotides to an antibody-DBCO PBS solution at a pH of 7.2 and a concentration of 1 mg/mL. Purify using ion-exchange chromatography.
Alternatively the oligonucleotide can be complexed with Protamine and then reacted with the antibody. Learn about bioconjugation to protamine in our related article.
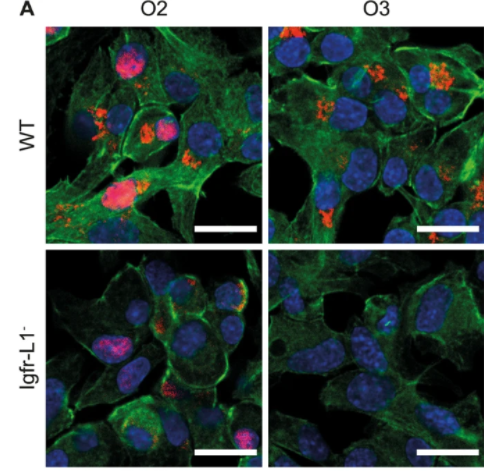
Functionalization of Water-Soluble Polymers
Water-soluble polymers can be functionalized to provide another alternative to antibody-drug conjugates. Here we present a method from Kim et. al (2019), in which they used azlactone-solubilized polymers. Read this article for more complete methods.
Explore conjugation kits for polymers, proteins, and antibodies, here.
Step 1. Synthesize and Functionalize PVDMA (poly(2-vinyl-4,4-dimethylazlactone) Polymer
Use RAFT (Reversible Addition Fragmentation chain Transfer) polymerization to synthesize a PVDMA homopolymer with a molecular weight of 13.1 kg/mol. Functionalize the polymer with water-soluble azlactone by treating it with 0.3 equivalents of mTEG (triethylene glycol monomethyl ether), the catalyst DBU (1,8-Diazabicyclo[5.4.0]undec-7-ene), and THF (Tetrahydrofuran) at 40°C to create PVDMA-mTEG.
Step 2. Conjugate the Polymer to the Protein Holo-transferrin (hTF)
Incubate PVDMA-mTEG with hTF in PBS (phosphate-buffered saline) with 15% v/v DMSO (dimethyl sulfoxide) at 4°C. Use SDS-Page to confirm that the bioconjugation reaction took place.
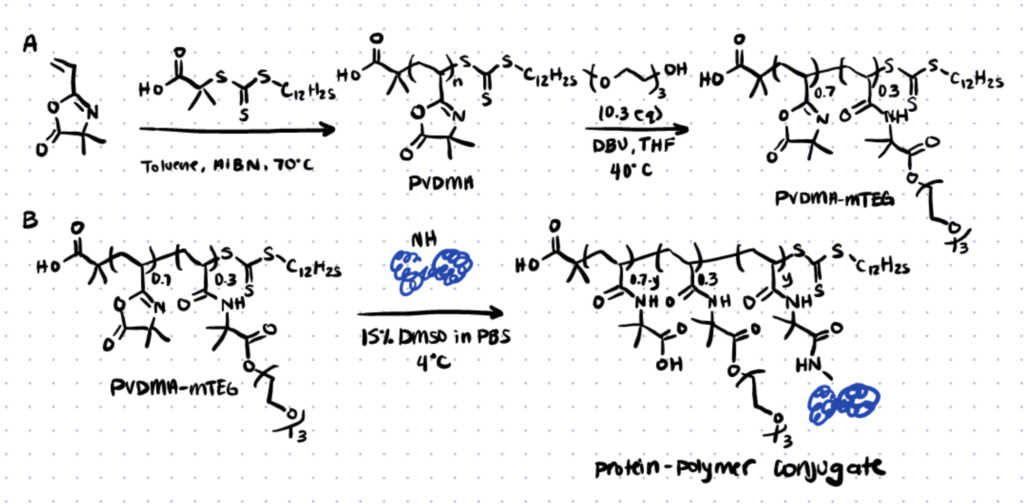
Applications of Water-Based Bioconjugation Reactions
Applications of water-based bioconjugation reactions include visualizing viruses and creating antibody-drug conjugates.
Application 1. Visualizing Viral Entry into Cells
Brandenburg et. al (2007) used fluorescent imaging to visualize the poliovirus entering live cells. They conjugated fluorescent dyes to label both the capsid and the viral RNA. The authors used their findings to hone models of viral cell entry.
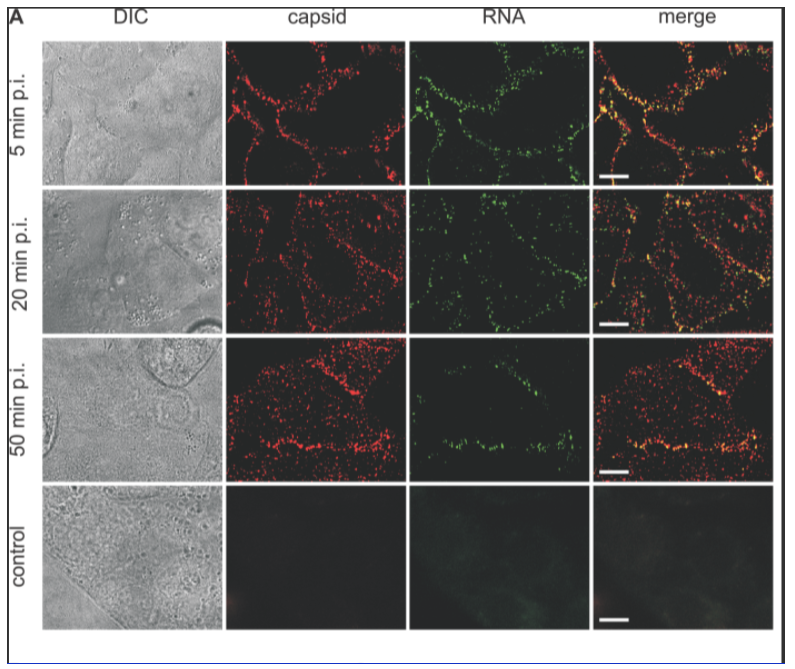
Application 2. Creation of Drug-Antibody Conjugates
Hamblett et. al (2004) conjugated drugs to antibodies at varying ratios, using the drug monomethyl auristatin E (MMAE) and the anti-CD30 monoclonal antibody cAC10. They used 2:1, 4:1, and 8:1 drug-to-antibody ratios and examined results both in vitro and in vivo. They found that for this set of specific drugs and antibodies, pharmacokinetics increased as the number of drugs per antibody decreased.
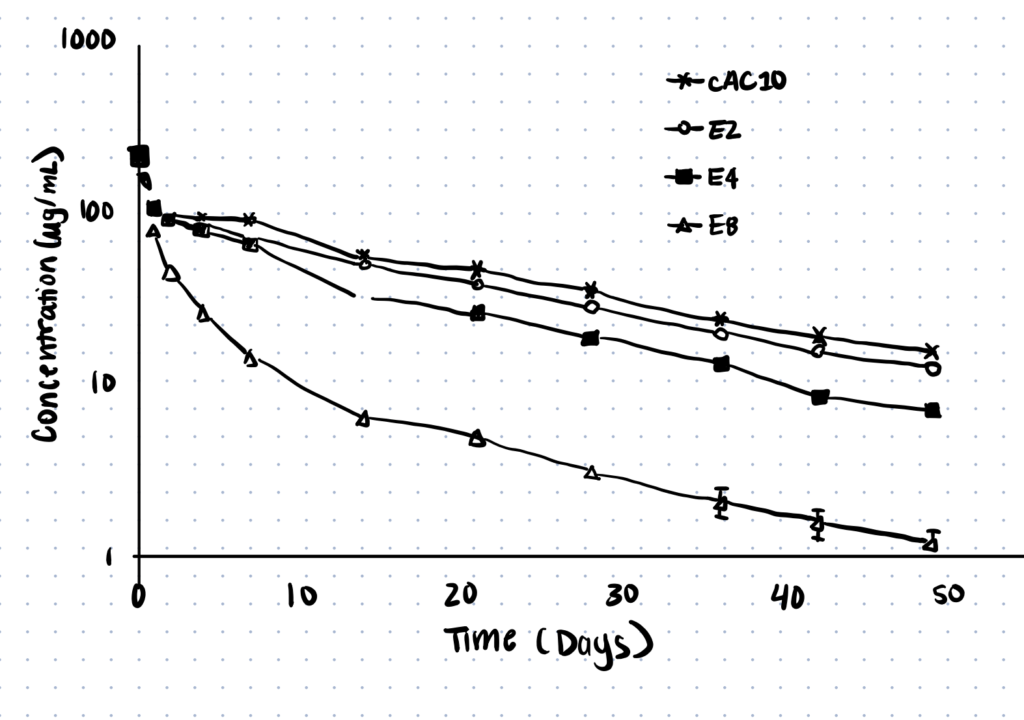