
Among the different types of nanomaterials available, magnetic nanoparticles (MNPs) have been the center of considerable attention for nanotechnology-based structures during the last two decades. As evidenced by an unprecedented increase in the number of research papers focusing on these materials, MNPs have revolutionized the fields of biotechnology, nanotechnology, and biomedicine by opening many doors to the discovery of ground-breaking therapeutic agents, including targeted delivery vehicles, electronics, imaging agents, and catalysts (Gloag et al., 2019; Gul et al., 2019; Ali et al., 2021; Zhang et al., 2019). In this article we will discuss protocols for magnetic nanoparticle bioconjugation and applications for the newly synthesized conjugates.
Magnetic nanoparticle bioconjugation techniques involve conjugation with proteins, polymers, and nucleic acids to improve their functionality. The newly synthesized structures can be used in applications such as magnetic cell separators, thermoelectric materials, and controlling water pollution.
What Are Magnetic Nanoparticles?
Magnetic nanoparticles have unique magnetic properties. Though challenging to synthesize due to their unstable nature, they possess a myriad of physicochemical properties suited for use in research and industry.
The term MNP covers a wide spectrum of nanoparticles (NPs) that possess the advantageous property of being magnetic in nature—and thus can be manipulated using magnetic fields. MNPs commonly consist of two components, a magnetic material and a functional chemical component, and are available in diverse architectures, including core-shell structures and Janus type structures.
MNPs possess unique physicochemical properties, such as high specific surface area, ease of functionalization, and chemical stability. General methods available for preparing MNPs include co-precipitation, mechanical milling, electrochemical deposition, thermal decomposition, microemulsion, polyol technique, and flame spray synthesis methods. Precision in the preparation and surface functionalization of the MNPs is critical as it may affect their physicochemical properties, stability, movement, and efficiency (Katz, 2020; Ali et al., 2021; Kush et al., 2021).
However, in synthesizing MNPs, there are challenges like dipole interactions, particle size and surface effects, size control, magnetic properties, and phase purity. Additionally, although the intrinsic properties of MNPs often make them superior to other types of NPs, they possess high surface energy and are often unstable upon oxidation–with the intrinsic tendency to aggregate, especially in physiological environments. Advances in MNP coating and/or synthesis methods will increase the potential for MNPs to be implemented in a wider range of applications (Ali et al., 2021; Kush et al., 2021).

Magnetic Nanoparticle Bioconjugation Techniques
Significant efforts have been dedicated to the development of innovative synthetics and coating approaches that make it easier to maintain the desired size, morphology, composition, and surface chemistry required for MNPs to fulfill their full potential and facilitate further translational applications.
Magnetic nanoparticle bioconjugation techniques include conjugation of nanoparticles to proteins, polyethylene glycol (PEG), and DNA via covalent amide bonding, chemical coprecipitation, and CuACC click reactions.
Bioconjugation Of Magnetic Nanoparticles To Proteins
Surface functionalization of the MNPs with protein offers various advantages over naked MNPs, including enhanced biocompatibility/biodegradability, less immunogenicity, and lower cytotoxicity. Recently, biotechnological applications of serum albumin (HSA and BSA) as a surface coating material for NPs were reported to improve biocompatibility, reduce unwanted adsorption of blood components, and increase the efficiency of tissue and cell targeting (Chubarov, 2022; Iwaki et al., 2012; Kalidasan et al., 2016).
Here’s a general method for magnetic nanoparticle bioconjugation of Fe3O4 MNP to BSA via covalent amide bonding for use in magnetic hyperthermia therapy. For more details refer to this article by Kalidasan et al. (2016).
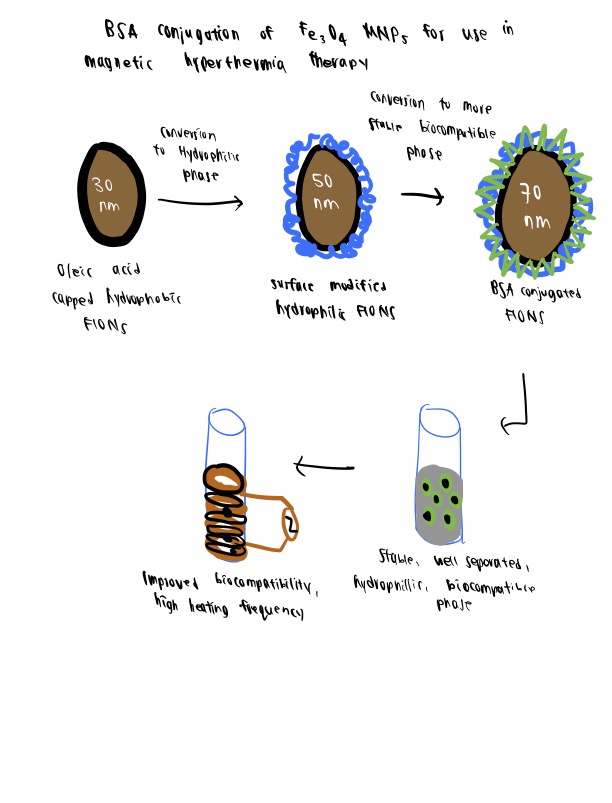
Step 1. Synthesis Of MNPs
In this study, the authors first synthesized the MNPs functionalized with oleic acid capping via the thermal decomposition method (280oC). Surface-functionalized MNPs show high magnetic saturation values.
Step 2. Conversion To Hydrophilic Phase
The newly synthesized MNPs are hydrophobic, due to the oleic acid capping, and have to be converted to a hydrophilic phase. The strategy of phase transfer is conducted using the ligand exchange strategy, in which the silane group of APTMS was exchanged for oleic moiety on the MNPs.
Step 3. Bioconjugation Of MNPs With Bsa
The carboxylic groups on the BSA are activated by the EDC method and a strong amide bond is formed between the carboxylic group of BSA and the exposed amino groups of the APTMS-capped MNPs. Improvements in biocompatibility, magnetic saturation values, colloidal stability, and heating efficiency make them ideal for magnetic hyperthermia therapy and further biomedical applications.
If conjugation with EDC interests you, consider reading our articles on EDC bioconjugation and antibody conjugation methods.
Bioconjugation Of Magnetic Nanoparticles To Polyethylene Glycol (PEG)
Surface modification of MNPs with polymers has long been employed as a promising strategy to improve some properties of the MNPs, including biocompatibility, biodegradability and chemical stability in a physiological environment. PEG—in particular—is one of the most commonly used coating materials for MNPs due to its hydrophilic property attributed to its hydration with bound water molecules (Amstad et al., 2009; Xie et al., 2007; Chekina et al., 2011). Additionally, PEG is also an FDA-approved excipient in numerous pharmaceutical formulations due to its biocompatibility, nonantigenic, protein-resistant properties, and uses in bioconjugation of PEGylated nanoparticles.
Here’s a general method of chemical coprecipitation technique for the magnetic nanoparticle bioconjugation of PEG-coated Fe3O4 MNPs. For more details refer to this article by Cheraghipour et al. (2013).

Step 1. Synthesis Of MNP-CAs
First, the MNPs are prepared through co-precipitation of Fe2+ and Fe3+ in an ammonia solution (80°C, 1 h, 9000 rpm). The MNPs are then incubated in a citric acid solution to yield MNPs stabilized with citric acid (MNP-CA) via the direct addition method, as described by Sahoo et al. (2005).
Step 2. Preparation of PEGylated MNP-CA
NH2-PEG is then covalently attached to the carboxylic moieties of citric acid anchored to the MNP-CAs via carbodiimide amidation chemistry.
Step 3. Characterization of the MNPs
The synthesized MNPs are characterized by various analytical techniques. It was found that the PEGylated MNP-CAs demonstrated high saturation magnetization, uniform particle size, good colloidal stability, and superparamagnetic behavior suitable for various biomedical applications.
Bioconjugation of Magnetic Nanoparticles to Nucleic Acids
DNA is one of the most prevalent linkers in bioconjugation chemistry due to its well-defined properties, programmability, cost-effectiveness, ease of modification, and ability to recognize a broad range of analytes. The conjugation of MNPs to DNA fragments serves as another unique example of the combination of magnetic properties and biological selectivity to improve the efficiency of DNA bioconjugates for the diagnosis/therapy of diseases (van der Sleen and Tych, 2021; Stephanopoulos, 2020).
Here’s a general method for magnetic nanoparticle bioconjugation using Fe3O4 MNP and DNA via CuAAC click reaction for use as a safe and efficient transfection agent. For more details refer to this article by Cutler et al. (2010).
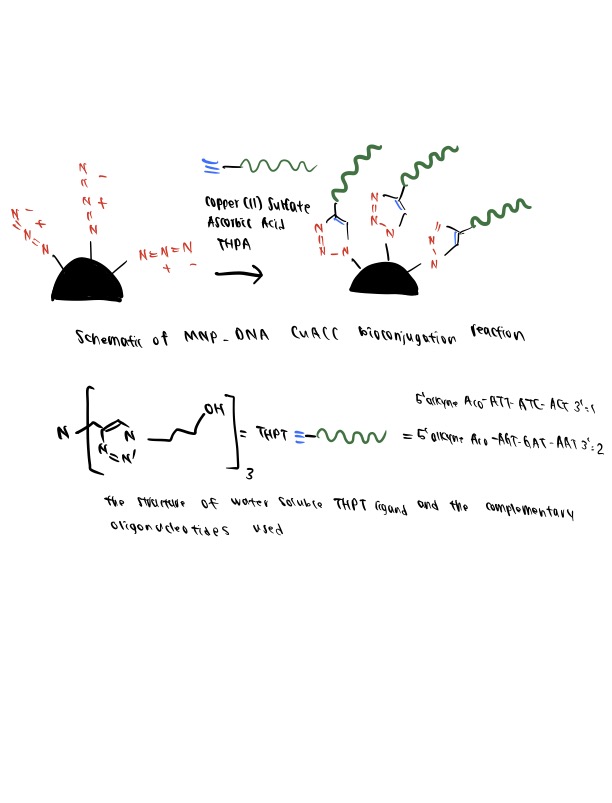
Step 1. Preparation Of Oligonucleotides
The authors prepare amine-functionalized oligonucleotide strands using a solution of alkyne-NHS ester, CuSO4, THPTA ligand, and ascorbic acid in DMSO. The presence of ascorbic acid and THPTA ligand in the reaction are necessary additions to prevent the well-known degradation effect of Cu(I) on oligonucleotides, as discussed by Kumar et al., 2007.
Step 2. Preparation Of Azide-Functionalized Mnps
Then, the MNPs are reacted with azido butyrate NHS ester in a DMSO solution overnight to yield azide-functionalized MNPs. The isolated MNPs can be resuspended in a PBS solution and kept at 4ºC for no more than a week.
Step 3. CuACC Bioconjugation Reaction
The azide-functionalized MNPs are added to the oligonucleotide-containing DMSO solution to induce the bioconjugation reaction. The catalytic elements are kept at optimal concentrations for producing stable conjugates, as reported in this study. The DNA-MNPs generated through this click chemistry, an example of orthogonal bioconjugation; exhibited improved properties, such as sharp melting transitions and high cellular uptake, indicating their dense functionalization.
What Are Magnetic Nanoparticles Used For?
The combination of their distinctive properties and the rich possibilities of coatings have given rise to the utilization of MNPs in various applications, with each potential application requiring different properties of the MNPs. Most commercially available MNPs are Fe3O4, attributable to their proven qualities, such as ease of synthesis, high chemical stability, cost-effectiveness, and biocompatibility (Nguyen et al., 2021; Ganapathe et al., 2020).
Applications of magnetic nanoparticles bioconjugates include their use as magnetic cell separators in the biomedical field, thermoelectric materials, and water purifiers to control environmental pollution.
Application 1. MNPs as Magnetic Cell Separators
Over the past several decades, MNPs have found broad applications in the biomedical field, ranging from the discovery of novel theranostic platforms and novel drug delivery systems—to performance-enhanced therapeutic/diagnostic alternatives (Gloag et al., 2019; Gul et al., 2019). One interesting example of biomedical utilization of MNP bioconjugates is the design of Fe3O4-SiO2-PMIDA for use in magnetically activated cell sorting.
In this study, MNPs are prepared via a three-step reaction, including fabrication of Fe3O4 MNPs via the co-precipitation method, the silanization of the magnetic core with TEOS, and the functionalization of Fe3O4-SiO2 MNPs by PMIDA. The fabricated MNPs show good performance following further testing through conjugation with antibodies, affording an efficient tool for the cell separation process with further potential to be applied to other areas of biomedical application.
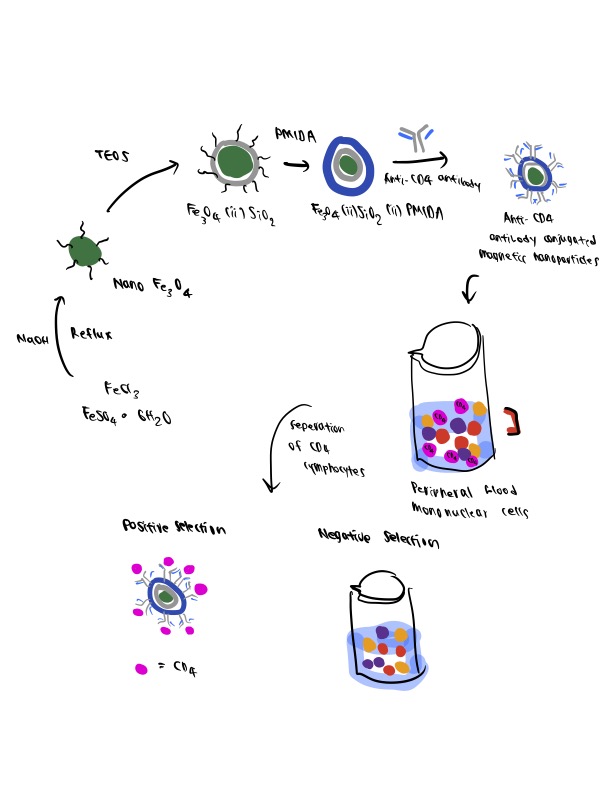
Looking for help maximizing cellular uptake of nanoparticles? Read our article, bioconjugation and cellular uptake: tips to maximize uptake of nanoparticles.
Application 2. MNPs as Thermoelectric Materials
Thermoelectric (TE) materials have received increasing attention in the design of thermal energy harvesting devices for waste-energy recycling and solid-state cooling. Recently, a breakthrough has been achieved by introducing MNPs into TE devices to improve their thermoelectric properties, due to the particle size-dependent superparamagnetic features and magnetocaloric effect of MNPs (Wu et al., 2022; Ma et al., 2020).
Here, Wu et al. (2022) demonstrated that the bioconjugation of Bi2Fe4O9 MNPs synthesized with hydrothermal methods into TE nanocomposites could effectively scatter low-energy carriers and improve the carrier quality. The synergistic effect of boundary and magnetic particle scattering enhances the Seebeck coefficient. This decreases thermal conductivity, and substantially increases the material’s ZT value. Compared to commercial nanocomposites, the result of this study brings new perspectives to the design of efficient and cost-effective TE devices.
Application 3. MNPs to Control Water Pollution
Unrestricted entry of wastewater into the environment has highlighted the importance of water purification systems providing effective contaminant filtration. MNPs offer excellent chemical demulsification performance, separation-capability, cost-effectiveness, and recycling potential that is advantageous over other wastewater treatment technologies (Farahbakhsh et al., 2021; Shukla et al., 2021).
In this study, Zhang et al. (2016) developed a novel class of MNPs for wastewater treatment that can effectively adsorb heavy metals and kill bacteria simultaneously. The fabricated M-QAC has a Fe3O4 core-shell structure surrounded by polyethyleneimine-derived QAC corona. When dispersed in water, the particles interact simultaneously with multiple contaminants—either pathogens or toxic metal cations/anions—in minutes. Additionally, the magnetic Fe3O4 core also allows for the separation/recycling of the M-QACs in a facile manner.
