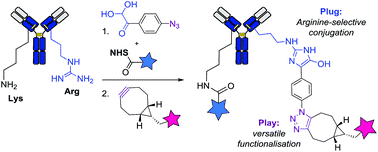
It all started in 1916 when Otto Diels first uncovered the chemoselective reactivity of α-dicarbonyl reagents with the guanidine group of arginine—opening up the possibility of arginine as another relevant nucleophilic amino acid for bioconjugation. Even though methodologies for selective arginine bioconjugation methods are rather scarce, these residues are of special interest for the modification of biomolecules.
Arginine bioconjugation techniques include the use of 4-azidophenyl glyoxal reagents, hapten-driven conjugation, and the use of NHS ester reagents.
Cysteine and tyrosine residues aren’t frequently located on protein surfaces and can be difficult to target in folded proteins. As such, bioconjugation methods targeting these residues frequently require complicated mutagenesis and refolding protocols. Given their abundance on protein surfaces due to their positive charge (pKa ~12), arginine now represents another relevant nucleophilic amino acid that is an attractive target for bioconjugation.
Arginine Bioconjugation Methods
Arginine bioconjugation strategies offer various advantages over the other more commonly targeted lysine, cysteine and tyrosine residues. Most of the described approaches for arginine bioconjugation chemistry are based on their reactivity with α-dicarbonyl compounds. However, several researchers have also exploited the residue using other conjugation reagents and protocols to further optimize the protocol—especially for the generation of homogenous adducts. We discuss several methods below.
Most proteins and antibodies have readily available amines and carboxyls. You can label proteins and antibodies with these conjugation kits to save time and improve consistency between experiments.
Method 1. Site-Selective Arginine Bioconjugation With 4-Azidophenyl Glyoxal
In 1968, Takahashi observed that phenylglyoxal reagent react rapidly and selectively with the guanidino group of arginine residues, resulting in the formation of a stable hydroxyimidazole ring. He later compared the reaction with two related reagents, glyoxal and methylglyoxal, and concluded that phenylglyoxal was the most selectively reactive reagent and provided better yield of conjugates. For more site-selective bioconjugation reactions, check out article about histidine bioconjugation.
In 2016, this reaction was utilized by Dawson et al. for the functionalization of two model proteins—namely the RNAseA and Lysozyme—by using commercially available 4-azido-phenylglyoxal (APG). More recently, Dovgan et al. (2018) demonstrated the applicability of APG as an efficient plug-and-play reagent for the selective functionalization of arginine residues in native antibodies, using trastuzumab as the model platform. The general protocol is described in the following section.
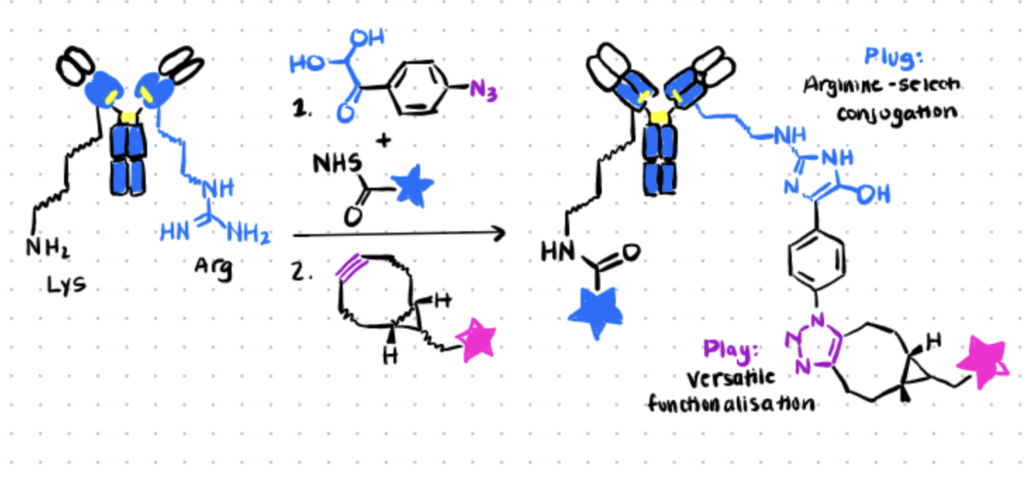
Step 1. Preparation Of Conjugates
To evaluate the rate of antibody modification, the authors first reacted trastuzumab with various amounts of APG in PBS. The reactions of APG with the α-amino groups involve the initial formation of Schiff bases. In order to stabilize the Schiff bases, APG will also react simultaneously to form a cyclic compound with a specific side-chain group having a reactive hydrogen atom—in this case, arginine.
Following gel filtration, the resulting conjugates were further functionalized with the fluorophore TAMRA-BCN, yielding the antibody-fluorophore conjugates T-TAMRA(R) for observation—in which they concluded that arginine acted as an APG scavenger during the first stage of antibody modification.
Step 2. Dual Orthogonal Antibody Functionalization
Further optimizing their research, the authors also performed antibody functionalization with two oligonucleotides via a plug-and-play approach with APG. Dual orthogonal modification of the antibody-azide conjugates with various payloads was achieved via the SPAAC method by conjugating trastuzumab with APG and Cy5-NHS on Lys and Arg residues, yielding the T-TAMRA(R)-Cy5(K) conjugates.
This experiment proved that the Arg/APG combination was orthogonal to Lys/NHS and that neither reaction was affecting the other. The resulting conjugates maintained the antibody’s recognition properties towards antigens and showed high stability in human plasma.
Method 2. Site-Selective Hapten-Driven Conjugation of Arginine
In the concept of hapten-driven covalent conjugation, a hapten that binds to a hapten-binding site of an antibody brings an electrophilic functionality close to a nucleophilic amino acid residue to trigger the formation of a covalent bond. Expanding the well-known concept of hapten-driven conjugation to Lys residues, Hwang et al. (2019) showed that replacing the Lys with an Arg residue affords a site-selective and stable ADC conjugate. The research followed two major steps.
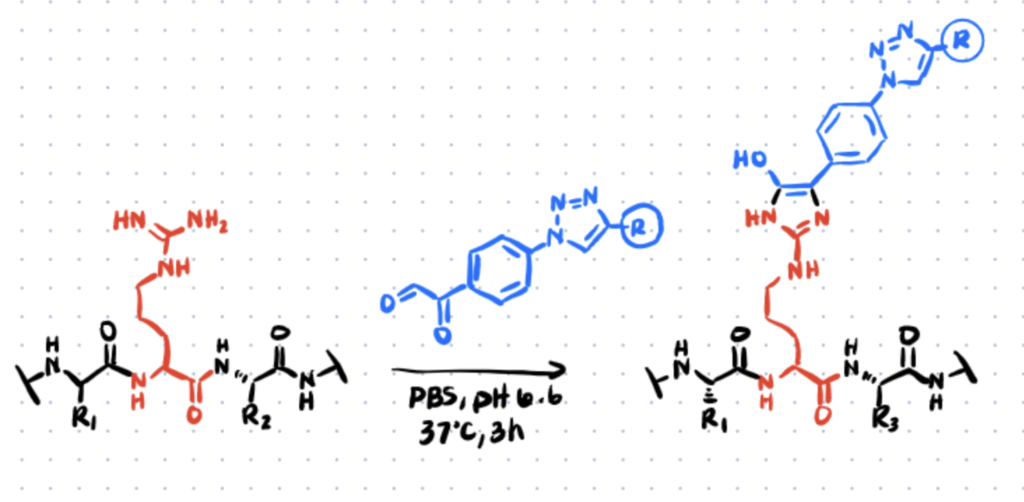
Hapten-driven covalent conjugation of a phenylglyoxal-triazole derivative to the reactive Arg residue with a modified antibody (Image Source: Cell Chemical Biology).
For other methods of bioconjugation using haptens, see our article on EDC bioconjugation.
Step 1. Confirm Orthogonal Arginine Bioconjugation First
X-ray crystallography of h38C2—a recombinant antibody generated using an aromatic hapten with β-diketone functionality—revealed a highly reactive arginine residue positioned at the bottom of a deep hydrophobic pocket that can be considered a hapten-like electrophile.
Similar to the previous method, the authors also utilized TAMRA to probe the arginine residue on h38C2 for site-specific conjugation. Following incubation at different molar ratios, SDS-PAGE analysis confirmed the formation of a hydroxy imidazole ring between the arginine and phenylglyoxal. The results obtained also suggested that arginine is the preferential site for covalent conjugation, as opposed to Lys residue.
Step 2. Generate Site-Selective Antibody Drug Conjugates via the Arginine
With conjugation efficiency, selectivity, and stability established, the authors next investigated the suitability of arginine:phenylglyoxal adducts for the assembly of site-selective ADCs. Using the same one-pot assembly as the previous step, the authors conducted a sequential conjugation reaction of the h38C2 with the phenylglyoxal derivative of TAMRA and an additional β-lactam hapten derivative of MMAF. Mass spectroscopy confirmed the presence of dually-labeled heterodimeric ADC conjugated with a single TAMRA and a single MMAF molecule (DVD-IgG1). According to the MS results, conjugation of h38C2 with arginine residues delivered similar conjugation efficiency with conjugation when compared with conjugation with Lys residues.
Method 3. Gas-Phase Arginine Bioconjugation using NHS Ester
Representing a great majority of modification reagents commercially available today, N-hydroxysuccinimide (NHS) ester bioconjugation is perhaps the most common activation chemistry for biomolecules. Originally, arginine was not regarded as a reactive site for NHS esters bioconjugation as the guanidino group tend to be protonated, and is therefore not nucleophilic, at a low pH range within which NHS esters are stable to hydrolysis in aqueous solution.
However, on the basis that both deprotonated arginine residues and NHS esters can coexist in gas-phase where pH is an irrelevant consideration, successful acetylation of arginine residues with NHS esters can be achieved. The reaction was by McGee et al. (2012) through ion/ion reactions—exhibiting a reactivity analogous to that observed with unprotonated primary amines such as the N-terminus or ε-amino group of lysine. The basic procedure below is described below.
Step 1. Preparation of the Reactions Mixes
The authors first performed acetylation of N-termini, methyl esterification of C-termini, and arginine hydrazinolysis to ensure that the arginines are in their protonated state when reacted with reagent anions. The authors used sulfo-NHS ester—a particularly useful gas-phase anionic reagent that can induce the formation of stable bonds due to the strong interaction of the sulfonate group with protonated sites on polypeptide cations (He et al., 2005; Han and McLuckey, 2005).
Step 2. Mass spectrometry
Mass spectroscopy analysis was conducted to provide a read-out of the bioconjugation reaction that occurred upon the reaction of the protonated arginine with the sulfo-NHS ester reagent. The results showed the facile displacement of NHS from NHS esters with concomitant covalent modification of the arginine residue, as confirmed by subsequent fragmentation.
Applications of Arginine Bioconjugation
Considered as one of the most versatile amino acids, arginine is essential for various key biological phenomena and is the only amino acid side chain that can maintain a positive charge in the hostile membrane environment (Brosnan and Rooyackers, 2013). Owing to their specific roles in membrane properties, high abundance in protein surface, and their abilities to interact with other amino acids and biomolecules, arginine bioconjugation methods have been employed by researchers for a broad range of applications because these conjugates are more homogenous and the chemistry is selective (Dovgan et al., 2018).
Applications of arginine bioconjugation include the development of antibody-drug conjugates, fabrication of 3D scaffolds, and as transporters to help enhance drug delivery mechanisms.
Trying to attach molecules together? You can explore conjugation kits to help you attach biomolecules together quickly and repeatably here.
Application 1. Development of Antibody-Drug Conjugates
Fueled by the mounting success of therapeutic and diagnostic monoclonal antibodies, substantial efforts have focused on the development of antibody-drug conjugates (ADCs) as vehicles to deliver potent cytotoxic payloads to specific cell types. As reviewed by Walsh et al. (2021), a wide range of methods for site-specific antibody bioconjugation have been developed. Although some of these processes have been successfully applied for the generation of homogeneous ADCs, most of them do not apply to native antibodies and require costly protein engineering techniques.
As previously discussed in the section on arginine bioconjugation with APG, Dovgan et al. (2018) reported the homogenous modification of trastuzumab arginine residues via condensation of its guanidine group—yielding antibody conjugates that maintained antigen selectivity and demonstrated high stability in human plasma. A more recent study of HER2-targeting ADCs based on Lys99 and Arg99 conjugation also show high precision, efficiency, and stability for delivery of hydrophilic therapeutic cargos.
Application 2. Fabrication of 3D Scaffolds
The click-chemistry era has generated a library of versatile reactions that are particularly advantageous for the design of sophisticated biomaterials—addressing problems commonly associated with natural biomaterials, particularly for tissue engineering and drug delivery systems. These reactions have also been utilized to induce powerful transformations to support structures such as hydrogels, 2D functionalized substrates, and 3D biomimetic scaffolds (Gopinathan and Noh, 2018)
One interesting example includes the preparation of biocompatible 3D collagen scaffolds from synthetic arginine-based poly(ester amide) polymers (Arg-PEAs) and chitosan derivatives by Udhayakumar et al. (2017). The authors chose arginine as a crosslinker and an active component to improve the physicochemical properties of the porous scaffold for two main reasons: a) arginine’s unique capacity to retain cationic charge over a wide range of pH and b) its role in wound healing. The overall results of the in silico, in vitro, and in vivo studies indicated that the scaffolds augmented cellular growth, promotes healing, and accelerates re-epithelialization and collagen deposition much faster than the scaffolds prepared with other amino acids as crosslinkers.
Application 3. Enhancing Drug Delivery Systems
Topical delivery offers obvious advantages over other routes of drug delivery. However, the complex lipid and cellular structure acting as permeability barriers within the skin pose a formidable challenge (Jeong et al., 2021).
Short arginine oligomers have been shown to efficiently cross a variety of biological barriers. According to Prochiantz (2000), the presence of guanidine groups in these peptides causes the spontaneous formation of inverted micelles—disrupting the biological membrane transiently. As observed by Rothbard et al. (2002), peptides conjugated with short arginine oligomers were able to enter cells more efficiently than the peptides containing no arginine residues.
A well-studied example includes the conjugation of a heptamer of arginine (R7) to enhance topical absorption of cyclosporin A (CsA)—a highly potent but poorly absorbed anti-inflammatory drug against a broad range of inflammatory skin disease. In contrast to native CsA that fails to penetrate the skin, topically applied R7–CsA was efficiently transported into cells in mouse and human skin. A similar study attaching arginine residue to Rh2, an anticancer agent with low oral bioavailability, also showed enhancement of efficiency of topical drug delivery to different tissues. For more information about drug delivery and bioconjugation check out our article about hydrazine bioconjugation and its applications.
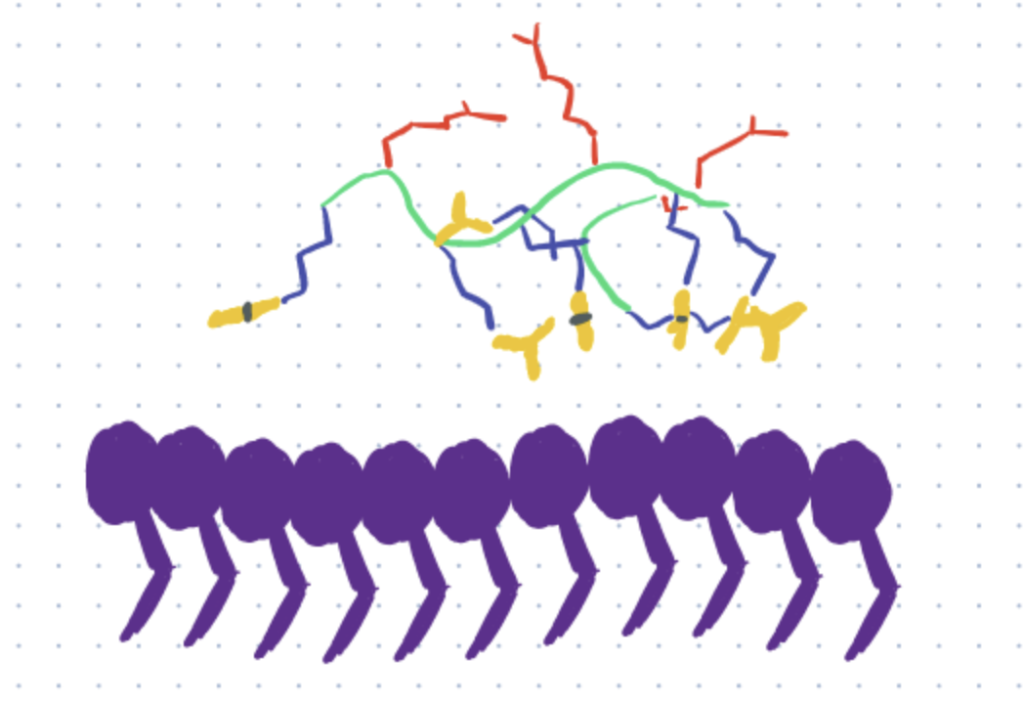