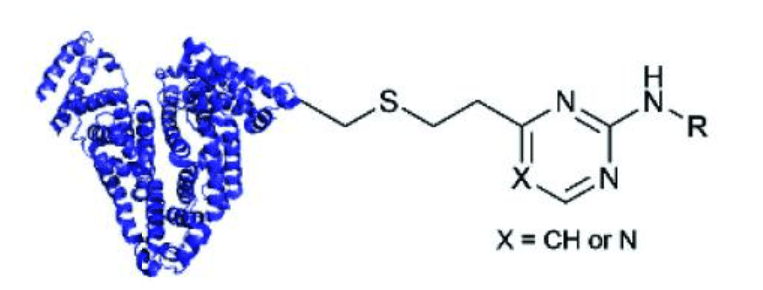
Site selectivity is a major obstacle in protein bioconjugation. One strategy is to introduce nonnative amino acid residues with unique functional groups into polypeptide chains. This allows for high selectivity but the process of introducing these amino acids is cumbersome. Another strategy is to target naturally occurring amino acids, such as cysteine. Cysteine is an ideal bioconjugation target due to its rarity and particularly nucleophilic thiol functional group, which can undergo a diverse range of reactions. In this article we’ll discuss cysteine bioconjugation techniques and applications.
Cysteine bioconjugation techniques involve either direct side-chain targeting, N-terminal targeting, conversion to other functional groups, or metal-mediated bioconjugation. To react with cysteine, common functional groups such as maleimides may be utilized or more specific techniques which utilize palladium catalysts, benzyl-isothiocyanates, or vinyl heteroarenes.
Related articles:
- You might enjoy this article on site-specific tyrosine bioconjugation techniques if you’re looking for a more selective amino acid to react with than cysteines
What is Cysteine Bioconjugation Used For?
Cysteine bioconjugation has applications in affinity tagging, bioimaging, therapeutic treatments, polymer chemistry, and fundamental protein studies.
Similar to other methods of protein bioconjugation, affinity tags, dyes, and fluorophores can be conjugated to cysteine residues for use in assays and bioimaging. Commons probes are biotin (learn more about biotinylation here) and PEG. You can even use cysteine bioconjugation for attaching quantum dots to proteins.
Cysteine bioconjugation is also frequently used in therapeutic protein conjugates, especially antibody-drug conjugates, or ADCs, which are currently being used in the treatment of HIV and cancer. It is well known that small therapeutic molecules and drugs can be cytotoxic. Therefore, the goal of ADCs is to utilize the antibody to target and deliver the drug to specific malignant cells.
Cysteine bioconjugation is also used in the formation of polymers, as well as in exploratory studies on proteins, biosynthetic pathways, and post-translational modifications (PTMs).
Thiols react readily and can be used for conjugation reactions. Papyrus Bio has a range of thiol-based conjugation kits. Explore thiol conjugation kits here.
Cysteine Bioconjugation Strategies
Cysteine bioconjugation strategies include direct side-chain targeting, N-terminal specific reactions, metal-mediated reactions, and reactions that first change cysteine to a different amino acid.
Direct Side-Chain Targeting
Generally, electrophiles are used to target cysteines’ thiol groups due to their nucleophilicity. Often this is done through a substitution reaction.
Haloalkyl reagents are a common electrophile class which target the thiol group in SN2 reactions. This includes iodoacetamide which bind to and cap cysteines prior to proteolysis. Haloalkyls can also be used to form stapled peptides, or link two cysteine residues together in an alpha helix to stabilize them. Unwanted reactions with other nucleophilic sites, such as N-termini and lysine and histidine side-chains are a concern.
Electrophiles can have substituents other than halogens as well. For example, 1,4-dinitroimidazoles target thiols in a unique cine substitution mechanism and perfluoroaromatic molecules use nucleophilic aromatic substitution.
Michael acceptors constitute a different, yet incredibly diverse electrophilic class of bioconjugation reagents. Michael acceptors undergo the Michael addition reaction, in which a nucleophilic site reacts with an unsaturated compound. In this case, the nucleophilic site is the thiol group, and common unsaturated compounds are 𝛼,𝛽-unsaturated carbonyls and unsaturated sulfones.
Maleimides are one of the most common 𝛼,𝛽-unsaturated carbonyls. They have fast kinetics, don’t usually form side products, and don’t need catalysts, but they can have stability issues. Not only are they used to add probes to cysteines, but they also can also add blocking groups and alkene functionality for further reactions. We’ve covered more information on bioconjugation of maleimides in our other article.
More recently, highly bio-orthogonal NGMs, or next generation malemides, have been utilized. They contain leaving groups and can be used to link multiple cysteine residues together. They also have more potential for selective cleavage, which can be used to deposit drugs in target cells. For other bioorthogonal reactions like azide bioconjugation reactions, read our article.
Maleimides may be one of the most commonly used Michael acceptors, but there is no shortage of them; each Michael acceptor has its own advantages and disadvantages. Examples of other Michael acceptors include aryl sulfones, 7-oxanobornadiene dicarboxylates, and allenamides.
Vinyl pyrimidines and other vinylheteroarenes are also used as Michael acceptors for cysteine bioconjugation. Divinylpyrimidine is used to bridge multiple cysteines together. Some electrophiles that are not Michael acceptors, such as isothiocyanates may undergo addition reactions, as well.
Alkenes and alkynes can also be used in radical reactions. First, a radical initiator such as UV light is used to turn the thiol group into a thiyl radical, which then reacts with the alkene or alkyne. These reactions are fast, they don’t require organic solvents, and they’re highly orthogonal, but many proteins will be cleaved in the presence of UV radiation and cannot be used.
This is similar to another UV-catalyzed reaction, photochemical alkylation, which has the benefit of reversibility. It is also important to note that alkynes are also capable of utilizing addition reactions due to their electrophilicity
Cysteines will naturally bond with each other and create disulfide bridges upon exposure to air. This can be an unreliable oxidation mechanism, though, since it’s slow and can result in protein dimers. Activated reagents like the Ellman Reagent and methanethiosulfonates allow for more controlled bond formation. These can be used in protein function studies, protein-polymer conjugation, and purification assays.
N-terminal Targeting
If a cysteine residue exists at the N-terminus, there are reactions and reagents that can be used to target it specifically, resulting in exceptional site selectivity. Native chemical ligation, or NCL, is a reaction between a thioester group and the thiol and amino groups at the N-terminus. This has been used to chemically synthesize proteins and peptides.
If an aldehyde or ketone is used to target the N-terminus instead, then a thiazolidine ring can be generated. This conjugate has applications as a cleavable ADC, but is unsuitable for diagnostic applications due to its instability.
2-cyanobenzothiazoles are another reagent that can be used to generate N-terminal thiazole rings. The disadvantage to all of these processes is that N-terminal cysteines often have to be engineered, as methionines are the typical residues at N-termini. For N-terminal bioconjugation techniques that don’t involve cysteine, read our article.
Most proteins and antibodies have readily available amines and carboxyls. You can label proteins and antibodies with these conjugation kits to save time and improve consistency between experiments.
.
2-cyanobenzothiazole is used to conjugate a target probe or molecule onto an N-terminal cysteine. A thiazole ring is generated in the process. Image Source: ChemBioChem.
Cysteine Thiol Conversions
To achieve site-specificity, or for exploratory studies, it’s sometimes useful to either convert cysteines into different amino acids or to convert the cysteine’ thiol into a different functional group. Both electrochemical bioconjugation and enzymatic bioconjugation can be utilized.
Hexamethylphosphorous (HMPT) can be used in a multi-step process to turn disulfide bridges into thioether linkages. Therefore, any material conjugated to cysteine via a disulfide bond can be made permanent, even in reducing conditions.
After NCL (native chemical ligation), metal or radical reagents can be used to convert cysteines to alanines. If cysteine analogs like 𝛽-mercapto-phenylalanine or penicillamine are used for the NCL instead of cysteine, then they can be converted to phenylalanine and valine, respectively.
Cysteine can also be converted to an alanine variant called dehydroalanine (Dha) with reagents like 2,5-dibromohexanediamides. Dha residues are electrophiles and can thus react with thiols in subsequent conjugation steps. This has led to many studies on post translational modifications and provides an alternative reaction mechanism to add probes to proteins.
Metal-mediated Bioconjugation
Cysteine has a natural affinity for many metals, however metal-mediated or catalyzed bioconjugation strategies present difficulties in vivo due to their cytotoxicity.
Metals can be used in a variety of different reactions. For example, as previously discussed, Nickel or palladium complexes can be used to reduce cysteine to alanine. Palladium complexes are also particularly useful for peptide stapling and for the generation of antibody drug conjugates (ADCs) due to their stability, speed, and chemoselectivity. We’ve discussed how to use palladium in bioconjugation of proteins, with protocols, in another article.
Gold can be used to mediate allene-thiol reactions, creating hydroxy vinyl thioethers. Ruthenium can catalyze olefin metathesis at S-allyl cysteine, a cysteine analog; this has been used to PEGylate and glycosylate proteins.
Another bioconjugation reaction that uses S-allyl cysteine is the Kirmse-Doyle reaction, which uses a rhodium carbenoid. Metals such as zinc can also be used as protecting groups on certain cysteine residues to allow for selective modification on the unprotected residues.
Cysteine Bioconjugation Applications
Applications of cysteine bioconjugation include creating antibody-drug conjugates, attaching fluorophores to proteins, and optimizing nanoparticle performance.
Application 1. Crosslinking Antibody-Drug Conjugates
Behrens et al., 2015, studied whether interchain cysteine crosslinking has an effect on the pharmacological properties of antibody-drug conjugates (ADCs). They created a novel bifunctional dibromomaleimide linker and conjugated it with two antibodies. They showed that this process leads to homogenous ADCs, which have better pharmacokinetic properties over conventional heterogeneous ADC mixtures, as well as increased efficacy and decreased toxicity. This is important, as ADCs have applications in cancer treatment.
Application 2. Testing Fluorophore Properties
Martin et al., 2019, compared fluorophore properties when conjugated to lysines or to cysteine-cysteine disulfide bridges. They discovered that the cysteine-bound fluorophores are more homogenous and stable, however, they suffer from fluorescence quenching. These methods provide more reproducible, consistent results.
Application 3. Optimizing Gold Nanoparticles
Cardoso-Avila et al., 2011, bioconjugated colloidal gold nanoparticles to cysteines and bovine serum albumin proteins. Conjugation made the nanoparticles more stable in saline conditions and therefore suitable for use in biological buffers. Gold nanoparticles have applications as optical biosensors and in pharmaceuticals.
Cysteine Bioconjugation Techniques
Cysteine bioconjugation techniques include the use of palladium reagents, benzyl isothiocyanates, and vinyl heteroarenes.
Organometallic Palladium Reagents For Cysteine Bioconjugation
In this section, we discuss a general technique for cysteine bioconjugation using organometallic palladium reagents. This is one of many protocols, collectively designed to show the utility of the palladium reagents in cysteine bioconjugation. For more details, including other specific reagents and protocols, please read this article.
Step 1. Synthesize and Purify Peptide P1
Synthesize peptides using Fmoc-SPPS (fluorenylmethoxycarbonyl solid phase peptide synthesis) chemistry using an HPLC (high-performance liquid chromatography) pump at 60°C with a 3-minute cycle per amino acid. After synthesis, deprotect the side-chains with a mixture of water, 1,2-ethanedithiol (EDT) and triisopropylsilane in trifluoroacetic acid (TFA). Triturate and lyophilize the peptides.
When needed, dissolve the peptides in guanidinium hydrochloride and purify with semi-preparative RP-HPLC (reverse-phase high-performance liquid chromatography).
Why do you need such a complicated mixture of solvents? Learn about challenges of bioconjugation in water in our related article.
Step 2. Synthesize Organometallic Palladium Reagent 1A-OTf
Add RuPhos (2-dicyclohexylphosphino-2′,6′-diisopropoxybiphenyl) and 4-tolyl trifluoromethanesulfonate to a cyclohexane solution. Add (COD)Pd(CH2SiMe3)2, then stir for 16 hours. Add pentane, freeze, filter and wash the precipitate, then dry under reduced pressure.
Step 3. Conjugate P1 to 1A-OTf
Add Peptide P1, water, an organic solvent comprised of water and acetonitrile, and Tris buffer together, then vortex. Add a solution of the palladium reagent 1A-OTf with more organic solvent, vortex, and leave for 5 minutes. Quench with 3-mercaptopropionic acid. Analyze results with LC-MS (liquid chromatography-mass spectrometry).
Cysteine Specific Bioconjugation with Benzyl Isothiocyanates
In this section, we discuss a general technique for cysteine bioconjugation using benzyl isothiocyanates. In the protocol, benzyl isothiocyanates are conjugated to the Fab arm of the trastuzumab antibody. For more details please read this article.
Step 1. Isolate the Fab Fragment of the Antibody Trastuzumab
Wash pepsin, a proteolytic enzyme, with a sodium acetate trihydrate digestion buffer, then add trastuzumab antibodies. Incubate, separate with a filter column, then wash with a digest buffer of phosphate, EDTA (ethylenediaminetetraacetic acid), and NaCl.
Activate papain, another proteolytic enzyme, with DTT (dithiothreitol) in the digest buffer. Wash without DTT, then add the previously digested trastuzumab antibodies. Incubate, separate with a filter column, and wash with BBS (borate-buffered saline).
Step 2. Reduce the Trastuzumab Fab Fragment
To break the disulfide bonds between cysteine residues, first dissolve TCEP (tris(2-carboxyethyl)phosphine) in water. Add the TCEP to the solution from Step 1 containing the Fab fragment and incubate. Exchange the old buffer with a new PBS (phosphate-buffered saline) buffer.
Step 3. Conjugate the Fab Fragment with Benzyl Isothiocyanate
Dissolve the benzyl isothiocyanate in DMSO (dimethyl sulphoxide) and dilute it with a PBS buffer. Add to the reduced Fab solution, incubate, and analyze with LC-MS (liquid chromatography-mass spectrometry).
Cysteine Bioconjugation with Vinyl Heteroarenes
In this section we discuss a general technique for cysteine bioconjugation with vinyl heteroarenes. In the specific protocol detailed, vinyl-pyrimidine is conjugated human serum albumin. For more details please read this article
Step 1. Reduce Human Serum Albumin Proteins
To eliminate disulfide bridges, add DTT (dithiothreitol) to solution of HSA (human serum albumin) in a PBS (phosphate-buffered saline) buffer. After incubation, ultracentrifuge into PBS.
Step 2. Conjugate HSA to Vinyl-pyrimidine
Add a solution of vinyl-pyrimidine Tris (tris(hydroxymethyl)aminomethane) HCl, and DMSO (dimethyl sulphoxide) to a solution of HSA in Tris HCl. Incubate and purify in a desalting column.