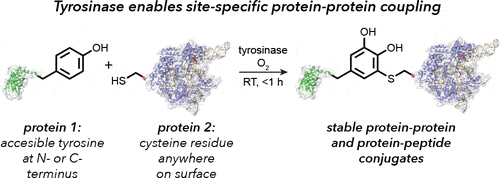
The early generation of bioconjugates was primarily synthesized through traditional chemical conjugation techniques. However, these techniques offer poor control over the site of modification — often resulting in heterogeneous mixtures and loss of the biological function of the target biomolecule. In this article, we provide a brief overview of enzymatic bioconjugation / enzyme-catalyzed bioconjugation techniques and their various applications in different fields. We also highlight several promising methods to achieve high yield and chemoselectivity.
Methods for enzyme bioconjugation and enzyme-catalyzed bioconjugation techniques include examples such as tyrinase mediated oxidative coupling of tyrosine tags onto proteins, site-specific bioconjugation through tyrosine-cysteine bond formation, and site-specific labeling of proteins using sortase.
With emerging evidence showing that site-specific conjugates exhibit superior stability, pharmacokinetics and potency in vivo, as compared to their heterogeneous counterparts, efforts have now shifted towards the development of new strategies to generate site-specific homogeneous constructs — for which the traditional bioconjugation methods are no longer adequate (Bai et al., 2020).
The use of enzymes as bioconjugation agents have increasingly gained scientific interest, particularly in the areas of biotechnology and pharmacology. The underlying specificity of enzymes and directed orientation of target molecules are what differentiates enzymatic bioconjugation for site-specific modification of biomolecules from other methods.
Related methods:
- To detect enzymatic bioconjugation was successful, you might need a high resolution assay. Check out our article on bioconjugation of quantum dots for this purpose.
What is Enzymatic Bioconjugation or Enzyme-Catalyzed Bioconjugation?
Enzymes are catalysts that play key roles in diverse post-translational modification of proteins in living organisms and industrial conversion processes. Their high specificity, rapid reaction rates, remarkable catalytic properties, biocompatibility with complex systems, and ability to function under mild reaction conditions make them excellent choices for protein labelling purposes. For example, enzyme-catalyzed bioconjugation of radiopharmaceuticals is one approach to developing theranostic (therapeutic and diagnostic) molecules.
Enzymatic bioconjugation or enzyme-catalyzed bioconjugation utilizes enzymes as signal generating reporters and/or catalysts to induce the formation of covalent bonds between biomolecules to ensure the maximum retention of activity of both.
Enzymatic bioconjugation offers advantages over non-specific reactions with regard to the conjugate’s properties and functions. These include enhanced biomolecule stability, enhanced binding efficiency, less variability in studies, and a higher level of binding.
Most proteins and antibodies have readily available amines and carboxyls. You can label proteins and antibodies with these conjugation kits to save time and improve consistency between experiments.
Enzymatically introduced bioconjugation occurs at the functional groups of amino acid side chains. Methods for enzymatic bioconjugation can be categorized into two main pathways (Zhang et al., 2018):
- Direct chemical modification of the amino acids side chains (cysteine, lysine, tyrosine, etc.) for functionalization with small tags, such as a fluorophore, biotin, glutaraldehyde, etc. as means of signal generation. Learn more about making a biotin-streptavidin conjugate in our related article.
- Introduction of non-natural amino acids (biorthogonal functional groups) into target biomolecules to provide reactive handles for more diverse and more specific subsequent conjugation reactions
Applications of Enzymatic Bioconjugation Techniques
Significant progress has been made in enzymatic bioconjugation techniques, and successfully applied in a vast number of applications.
Applications of enzymatic bioconjugation techniques include the generation of therapeutic protein-protein bioconjugates, bioconjugation to nanoparticles for drug delivery and the preparation of hydrogels with natural polymers under mild conditions.
Enzymatic Bioconjugation to Nanoparticles
Enzymes have great potential as therapeutic agents. However, due to several problems, the application of enzymes in clinical practice has been limited. Additionally, bioconjugation of enzymes with traditional methods is confounded by several common bioconjugation problems. We cover problems and solutions in the linked article.
Recent developments have reported the potential use of nanoparticles as carriers of enzyme drugs for convenient handling, enhanced stability and retention of functionality. The growing utility and availability of biocompatible nanoparticles, along with the concomitant necessity to functionalize them with a biological component in a controlled manner suggests enzyme-nanoparticle conjugation as the next logical step for optimizing therapeutic enzymes (Vertegel et al., 2011). For non-enzymatic methods for bioconjugation of nanoparticles, read our related article.
Other than application in the therapeutic field, nanoparticles have also been employed in a broad range of other applications — including cellular imaging, energy harvesting, fluorescent labeling, chemical catalysis, and many more.
Enzyme-catalyzed bioconjugation to nanoparticles offer specificity, increased stability, functionality, and directed orientation for modification of nanoparticles.
Several enzymes of interest for biocatalysis of nanoparticles include arylmalonate decarboxylase and glutamate racemase (Walper et al., 2015).
Enzymatic Bioconjugation for the Preparation of Hydrogels
The development of bioactive hydrogels has recently received attention because their mesh structures represent good mimics to extracellular matrices and offer the possibility to entrap bioactive molecules such as growth factors.
Enzyme-catalyzed bioconjugation has proven to be an easy, efficient, cell-friendly, substrate-specific alternative for the preparation of hydrogels (Teixeiria et al., 2012)
The mild conditions used in enzymatic reactions (moderate temperature, neutral pH, aqueous solution, etc.) provides a suitable environment to conjugate natural polymers that cannot withstand harsh chemical conditions to form hydrogels.
Using enzymes can also help avoid unwanted side reactions, toxicity, and possible loss of bioactivity that are commonly observed in hydrogels obtained with UV-mediated photo-crosslinking or physical crosslinking. A more comprehensive overview of recent advancements in enzyme-hydrogel bio conjugations for various applications can be found here.
Protein-Protein Bioconjugation
Protein-protein and/or protein-peptide conjugates are two of the most used applications for bioconjugation. They can serve as valuable constructs for vaccines, immunotherapy agents, signaling peptides with enhanced circulation properties, and targeted delivery vehicles (Rashidian et al., 2013).
We’ve discussed multiple protein-protein conjugation techniques here and provided step-by-step methods.
Trying to attach molecules together? You can explore conjugation kits to help you attach biomolecules together quickly and repeatably here.
Methods for Enzyme-Catalyzed Bioconjugation
Promising methods have been actively developed for selective enzymatic bioconjugation. In particular, Here, we highlight several novel methods of enzymatic conjugation that would enhance existing, well-established strategies in your lab by increasing efficiency and yield.
Methods for enzyme-catalyzed bioconjugation include the use of tyrosinase to generate reactive O-quinones and site-specific labeling with sortase by utilizing a sortase recognition motif.
Tyrosinase Mediated Oxidative Coupling Of Tyrosine Tags On Peptides And Proteins
A recent breakthrough in this chemistry by Marmelstein et al. (2020) involves the use of tyrosine amino acids with a simple phenol tag appended to cargo molecules to generate o-quinones for spontaneous conjugation with exogenous amine nucleophiles.
This method provides an appealing approach for protein bioconjugation because it avoids the increased complexity and lower expression yields that can be associated with unnatural amino acid incorporation. For a more detailed guide, read this paper. We’ve covered tyrosine bioconjugation in more detail in another article.
Step 1. Preparation Of Constructs With Tyrosine-containing Tags
Chemoenzymatic attachment of the -GGY tag encoded with tyrosine residues to the C-terminus of a single chain variable fragment on the cargo molecule.
Step 2. Tyrosinase-catalyzed Oxidation Of The Phenol Tag
Use activated tyrosinase (phosphate buffer, pH 6.5, 4-23°C) to catalyze the oxidation of the tyrosine residues on the phenol tag to form respective o-quinones, with the concomitant reduction of oxygen to water.
Step 3. Spontaneous Conjugation With Side Chains Of The Target Protein
The reactive o-quinone can further react non-enzymatically to form covalent protein-protein cross links via 1,4-additions with the side chains of lysine, tyrosine, histidine, and cysteine residues on the target protein (depending on their abundance and accessibility) to yield a conjugate.
Site-specific Bioconjugation Through Enzyme-catalyzed Tyrosine–cysteine Bond Formation
Another method reported by Lobba et al. (2020) provides an alternative for tyrosinase-mediated bioconjugation. The authors observed that cysteine thiols can participate readily with o-quinones at low equivalencies.
This chemistry offers a much simpler method for the covalent coupling of proteins and peptides using only native amino acid side chains. In the following section, we discuss a general process for site-specific bioconjugation through the enzyme-catalyzed tyrosine-cysteine bond formation. More information can be found in this paper by Lobba et al., 2020.
You might also consider using electrochemical bioconjugation techniques for tyrosine-specific conjugation.
Step 1. Oxidation Of Phenols To Yield O-quinone
As described previously, utilize tyrosinase to catalyze the oxidation of tyrosine residues to yield o-quinones.
Step 2. Addition Of Cysteine Thiols on A Target Protein To O-quinone
Surface-exposed cysteine thiols can readily form a covalent bond with the o-quinone on the 5-position of the cargo molecule to produce a product predominantly in its catechol form. Under optimal conditions ( pH 6.5-7.0, 4-23°C), the conjugation reaction can occur in 30 minutes to 1 hour.
Site-specific Labeling of Proteins Using Sortase Mediated Reactions
Sortases possess transpeptidase activity to break a peptide bond between the threonine and glycine residue of the sortase recognition sequence. It is especially useful for labeling because it will work on almost any protein bearing the respective sortase recognition motif, as reported by Popp and Ploegh in this paper. You can find additional methods for N-terminal bioconjugation of proteins in our related article.
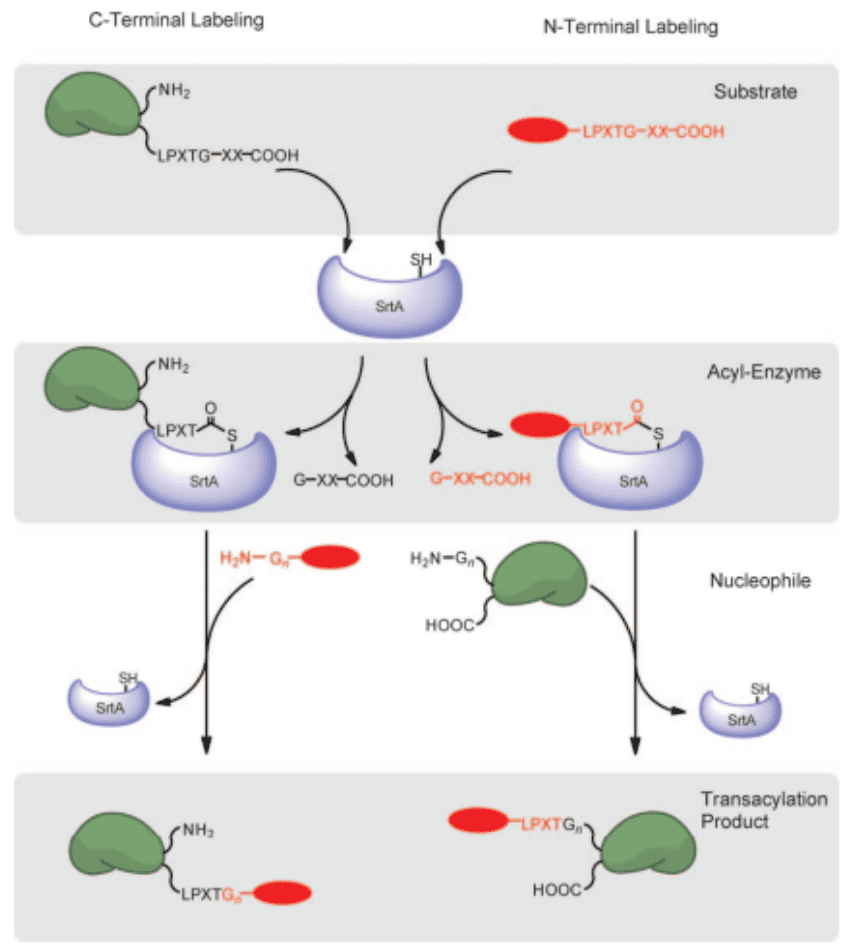
Step 1. Incorporation Of Sortase-recognition Motif On A Protein and A Labeling Molecule
Incorporate a sortase-recognition motif on the C-terminus of a protein using standard molecular cloning protocols.
Step 2. Generation Of Thioacyl Intermediates
Utilize Sortase to recognize the specific motifs on the protein and generate the thioacyl intermediate.
Step 3. Formation Of A New Peptide Bond
This intermediate then undergoes nucleophilic attack by the N-terminus of a target molecule of your choosing. This could be a label, a drug, or another protein.