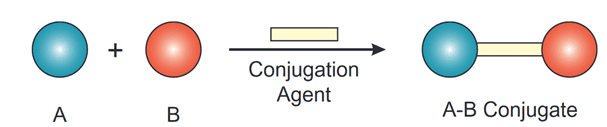
Different bioconjugation characterization techniques include separation techniques such as high-pressure liquid chromatography, scattering techniques such as dynamic light scattering, microscopy techniques such as near-field scanning optical microscopy, and spectroscopic techniques such as mass spectroscopy.
Bioconjugation characterization is necessary to ensure that the intended function and structure of the bioconjugates are optimized. HPLC can be used to purify, select, and quantify bioconjugate products, DLS to determine the zeta potential in concentrated or diluted samples, NSOM to visually observe the molecular structure of bioconjugates, and MS to determine bioconjugate composition.
What Is Bioconjugation?
Bioconjugation involves chemical reactions that combine two molecules–one of biological origin and the other as a synthetic fragment—through covalent bonds to form a bioconjugate.
Designing a bioconjugate starts by analyzing the eventual function it is intended to carry out and visualizing the desired features in the final complexes and conditions where they will be used. Despite the wide range of options to create a conjugate, matching the correct cross-linking agents, or reactive groups with the accessible functional groups on the components to be conjugated, is key to building a successful and active bioconjugate complex.

Bioconjugates can be used for many applications and stratified into six major areas:
- Assay and quantification
- Detection, tracking, and imaging
- Purification, capture, and scavenging
- Catalysis and chemical modification
- Therapeutics and in vivo diagnostics
- Vaccines and immune modulation
Understanding common bioconjugation problems and their solutions is necessary to ensure the efficacy of bioconjugates in practice. Each case has to be carefully designed and optimized for its intended use. The main advantage of utilizing bioconjugates is that they have attributes not found among naturally occurring reactions. In addition, many are also not exclusive to a single technology and can be successfully transferred to an interdisciplinary array of applications (Sapsford et al., 2011).
Bioconjugation Characterization Techniques
Bioconjugation characterization techniques include high-pressure liquid chromatography, dynamic light scattering, near-field scanning optical microscopy, and mass spectroscopy.
Technique 1. High-Pressure Liquid Chromatography
High-pressure liquid chromatography (HPLC) is an analytical technique used to separate, identify and quantify components of a mixture. It is the improvement of column liquid chromatography, where the solvent is forced through the column under high pressures instead of falling under gravity.
Generally, HPLC instrumentation consists of five components: a pump, injector, column, detector, and recorder. A solvent mixture is resolved using column chromatography, and the identification and quantification of the chemical features through spectroscopy.
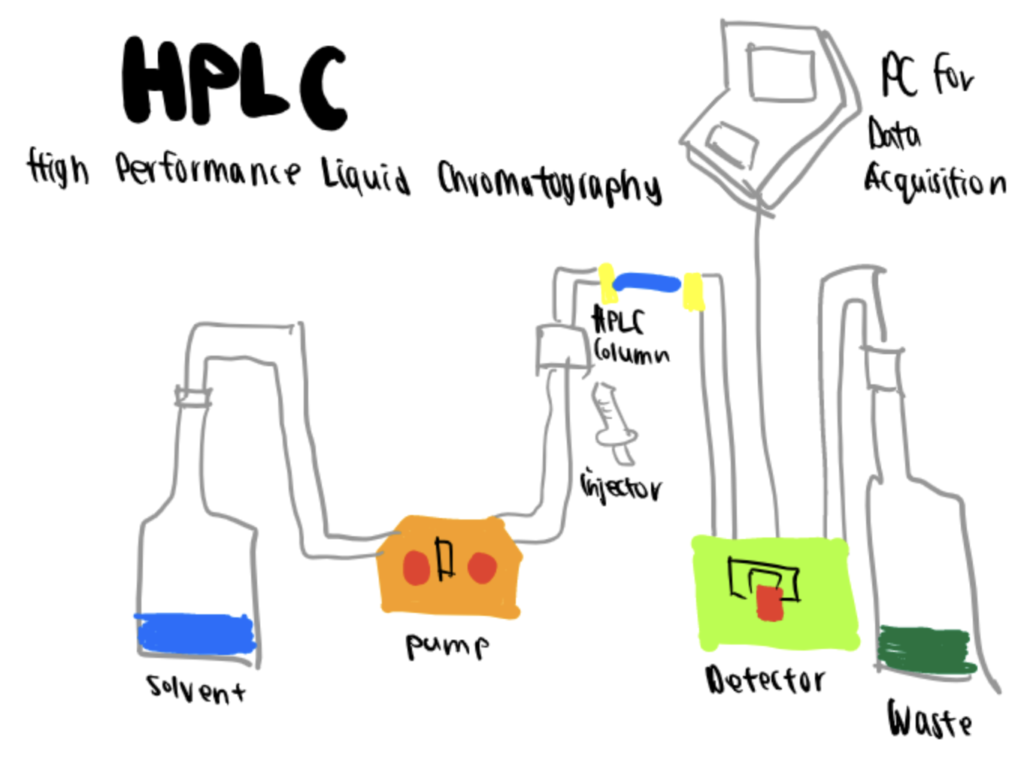
Essentially, in this method, the purification of the sample takes place in a separation column between a stationary (solid or liquid layer supported on an inert solid) and a mobile (liquid) phase that are immiscible with each other. The components with better affinity to the stationary phase will move through slower than those with less affinity. These speed differences will produce the separation of the components of the mixture. As they exit the column, they are detected and further analyzed (Aryal, 2022).
There are four types of HPLC, and the difference lies in the composition of the mobile and stationary phases:
- Normal phase: column packing is polar, and the mobile phase is non-polar.Reverse phase: column packing is non-polar, and the mobile phase consists of water and a miscible solvent
- Ion exchange: column packing has ionic groups, and the mobile phase is a buffer
- Size exclusion: the mixture is dispersed into the pores of a porous medium, and they separate as a result of their relative size to the pore size
In addition to being an efficient, accurate, and quick method, HPLC can be applied in almost all areas of chemistry, biochemistry, and pharmacy due to its precision in the identification and quantification of the components from the chromatogram analysis obtained at the end of the process.
Unavoidably, there are some drawbacks or limitations, such as the cost of reactives, the complexity of the method, coelution, and adsorbed compounds. However, HPLC and its variants have become one of the most commonly used worldwide due to its applicability to diverse analyte types, including reaction products and polymers formed through bioconjugation in water.
Technique 2. Dynamic Light Scattering
Dynamic Light Scattering (DLS), also known as photon correlation spectroscopy (PCS) and quasi-elastic light scattering (QELS), is a technique to determine particle sizes from 0.3 to 10000 nm in suspensions and emulsions. It can measure molecular weight, concentration, and zeta potential in highly concentrated or diluted samples, including the potential to characterize products formed through the bioconjugation of nanoparticles.
Zeta potential, the abbreviation of electrokinetic potential in colloidal systems, is the electric potential difference between the mobile dispersion medium and the stationary layer of the dispersion medium attached to the dispersed particle (Bruschi, 2015). In other words, if the particle moves, the electrical double layer moves with it along the so-called slipping plane. The Zeta potential is the electric potential at this slipping plane.
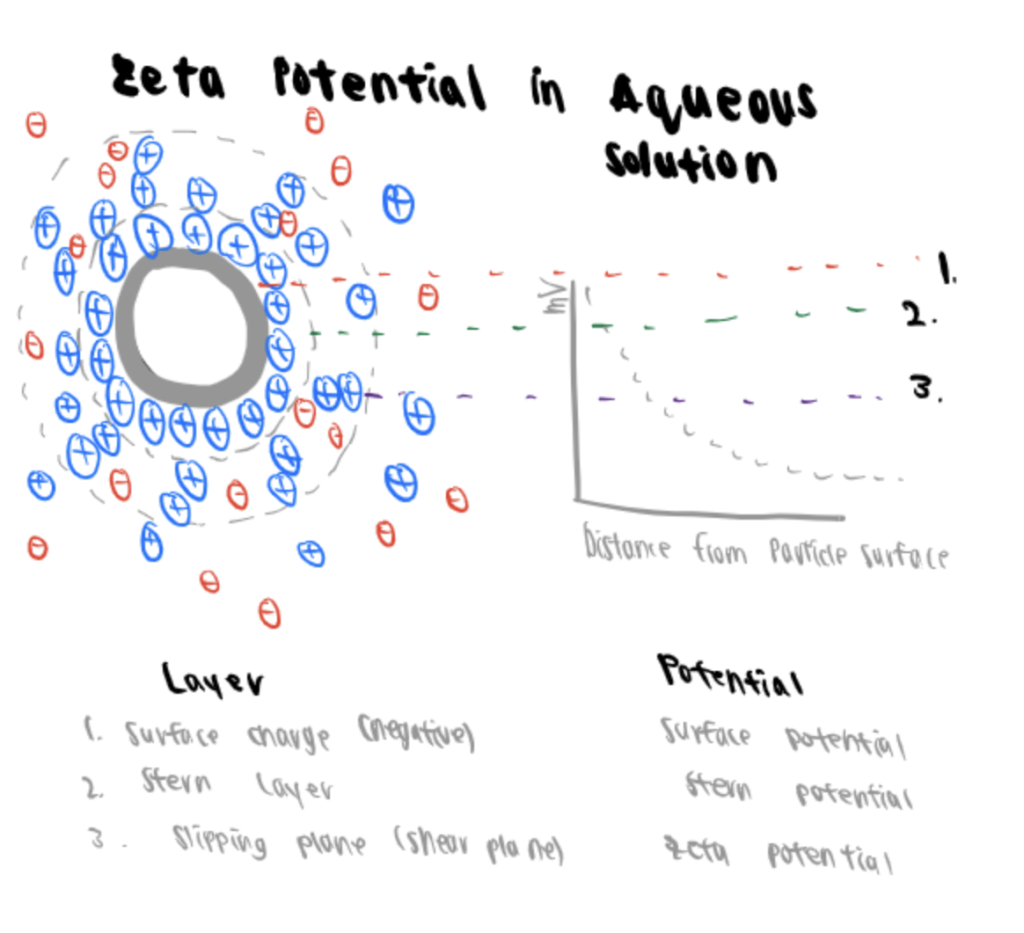
This potential is relevant because it can indicate the stability of a dispersion or emulsion. Zeta potential is determined via DLS technology with the same power spectrum methodology applied to measure nanoparticles.
DLS is based on the Brownian motion of particles that determines that smaller particles move faster than larger ones in a liquid. The particles are illuminated with a laser, and the light scattered by them holds information about the diffusion speed and the size distribution.
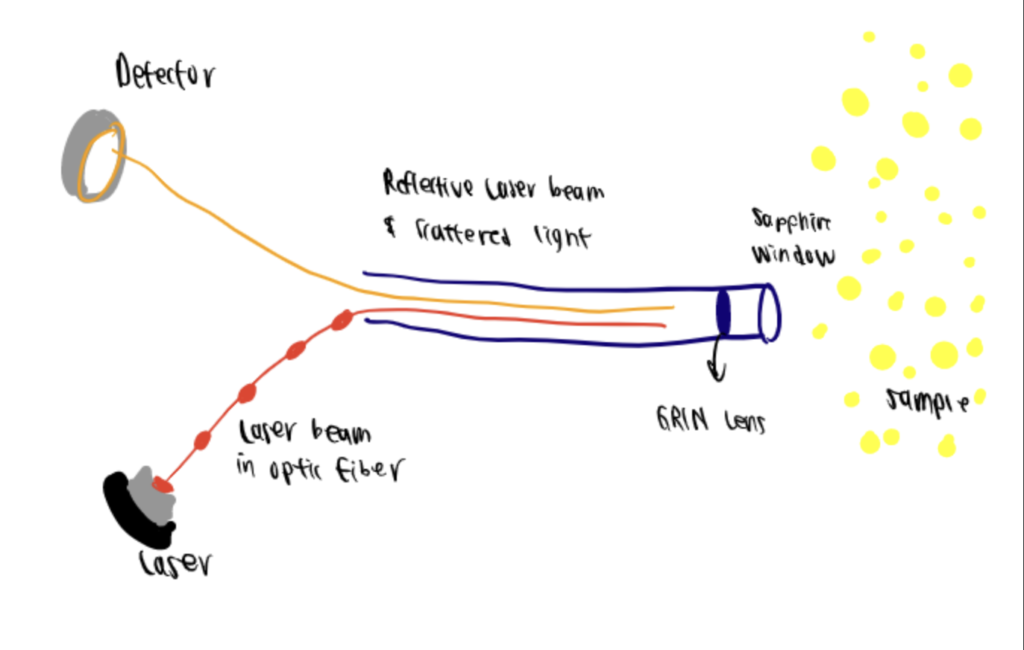
Finally, the signal detected is evaluated by PCS or through a power spectrum analysis, which are reliable because of their sensitivity, accuracy, and resolution.
Technique 3. Near-Field Scanning Optical Microscopy
Near-field scanning optical microscopy (NSOM) is a technique that enables non-destructive imaging of macromolecular assemblies such as lipid microdomains and receptor clusters within their native environment. Through NSOM, the images generated show the actual sizes of the assemblies, and it was developed to be utilized in both air and aqueous environments using nonionizing and visible radiation.

To briefly describe the principle behind NSOM, visible light is incidentally on a completely opaque screen with a subwavelength aperture. The radiation emanated through is collimated or aligned to the aperture size in the near-field regime. Still, in the far-field regime, the effect of diffraction is evidenced as a deviation that no longer duplicates the geometrical image of the aperture. In this sense, a cell membrane can be placed in the near-field, the light source can be scanned over it, and the detected light can then be used to generate a high-resolution image, which largely depends upon the aperture size rather than the wavelength (Lewis et al., 1986). More recently, one application of NSOM is to ensure that a bioconjugation process is accomplished and the products are viable (Zhang, 2006).
Technique 4. Mass Spectroscopy
Mass spectrometry (MS) is an analytical technique used in bioconjugation characterization that provides quantitative and qualitative information regarding the composition and size of macromolecules such as proteins, oligonucleotides, oligosaccharides, lipids, and even amino acids such as during arginine bioconjugation.
This technique determines the characteristic fragments or ions that appear by organic molecule breakdown by bombarding the analyzed compound with electrons to generate positively charged ions. These cations are fragmented, accelerated, and deflected in a circular path using a magnetic field directed at a photographic plate detector according to their mass-to-charge ratio. Each ion is recorded in the form of peak intensity on a mass spectra plot.

One of the most significant advantages of MS is its open-configuration format. The applications are expansive and can range from proteomics, metabolomics, and environmental analysis to pharmacy, forensic science, and medical research.
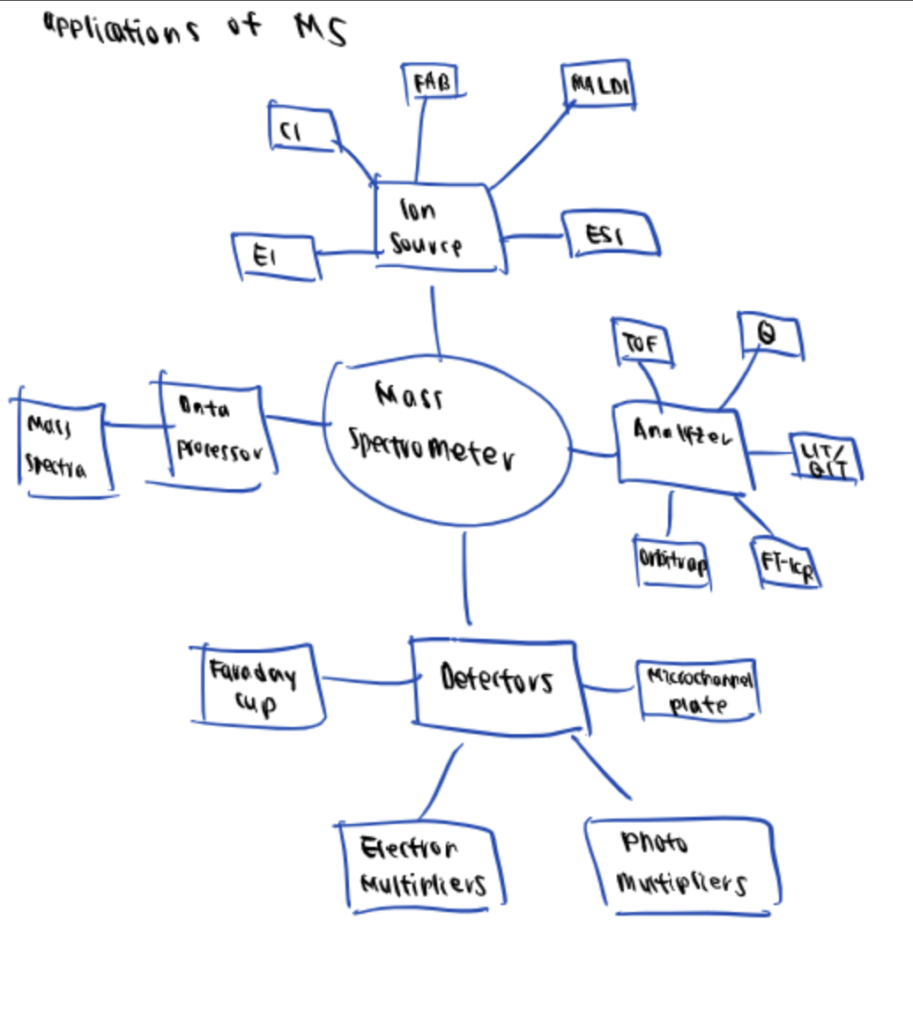
Summary Of Bioconjugation Characterization Techniques
Depending on the molecules and bioconjugates you are working with and would like to characterize, different techniques such as HPLC, MS, DLS, and NSOM can be leveraged.
Molecule You Are Working With | What You Want to Characterize | Suggested Technique |
Polymer, protein, antibody bioconjugates | Size | High-pressure liquid chromatography |
Polymer, protein, antibody bioconjugates | Composition | Mass spectroscopy |
Bioconjugated nanoparticles | Size, zeta potential | Dynamic light scattering |
Quantum dot bioconjugated protein | Bioconjugation process | Near-field scanning optical microscopy |