
Carbodiimides such as 1-Ethyl-3-[3-dimethylaminopropyl]carbodiimide hydrochloride (EDC) are heterobifunctional coupling reagents that have been mainly used for coupling carboxyl and amine groups since the 1970s. EDC is now commonly used with N-hydroxysuccinimide (NHS) or its water-soluble analog, Sulfo-NHS, to activate carboxyl functional groups to form amine-reactive intermediates that spontaneously reacts with primary amines to form amide bonds. Ultimately, EDC bioconjugation is an effective means of creating crosslinks to connect polymers and has numerous implications for biochemical conjugation assays.
EDC bioconjugation protocols involve first equilibrating reagents to room temperature, then adding EDC and stirring, then adding sulfo-NHS, leaving the reaction to complete over a few hours, and finally quenching the reaction with excess amine containing molecules.
What Is The EDC Crosslinker?
The EDC crosslinker activates carboxyl groups to form an unstable O-acylisourea intermediate which reacts with primary amines to form amide bonds. This reaction can be stabilized with the addition of NHS or Sulfo-NHS.
EDC is the most common zero-length crosslinker used in biochemical conjugations. It is well known for its efficiency in coupling two molecules of proteins, proteins and peptides, proteins and oligonucleotides, and proteins with small molecules (Wickramathilaka and Tao, 2019). It activates carboxyl groups, enabling them to couple with primary amines directly through covalent bonding similar to C-terminal bioconjugation. Some advantages of this crosslinker include the water-solubility of its byproducts as bioconjugation reactions in water allow for more thermodynamically relevant conditions that aid in protein folding (Gilles et al., 1990).
These attributes have promoted several applications, and some will be addressed further at the end of this article. Still, in a general view, they are related to protein bioconjugation, such as conjugation of carboxyl and amino groups among peptides and proteins and hapten coupling to immunogenic carrier proteins. In addition, activation of some molecules can also be carried out with this method; some examples are NHS-activated amine-reactive labeling compounds or nanoparticles of amine-reactive Sulfo-NHS esters.
Two main conjugation strategies have been carried on with EDC. The first one is a direct reaction to the target groups mentioned above, and in the second one, NHS or Sulfo-NHS can be added.
What Is The EDC Coupling Mechanism?
EDC reacts with carboxylic acid groups to form an active O-acylisourea intermediate easily displaced by nucleophilic attack from primary amino groups in the reaction mixture. The primary amine forms an amide bond with the original carboxyl group. An EDC byproduct is released as a soluble urea derivative.
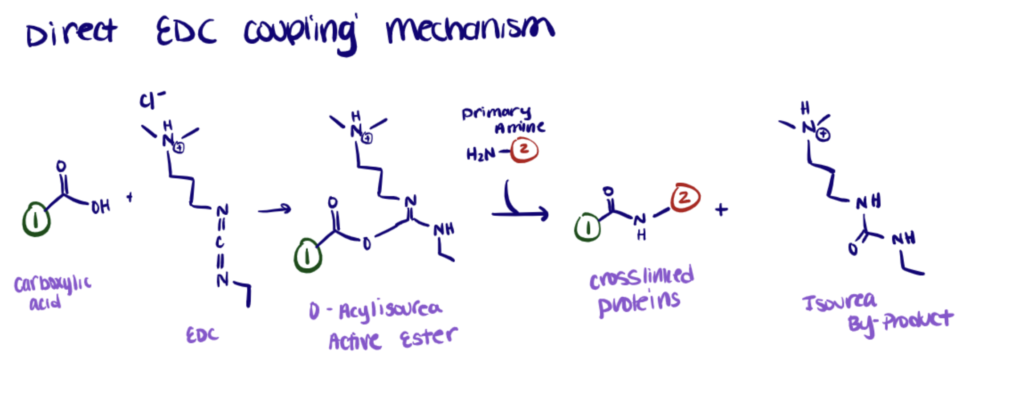
How Is EDC/NHS Coupling Different From EDC Coupling?
NHS, or Sulfo-NHS, is used to improve the efficiency of EDC bioconjugation and to stabilize the amine-reactive intermediate (O-acylisourea).
The addition of sulfo-NHS stabilizes the amine-reactive intermediate by converting it to an amine-reactive sulfo-NHS ester so that the efficiency of EDC-mediated coupling reactions increases.
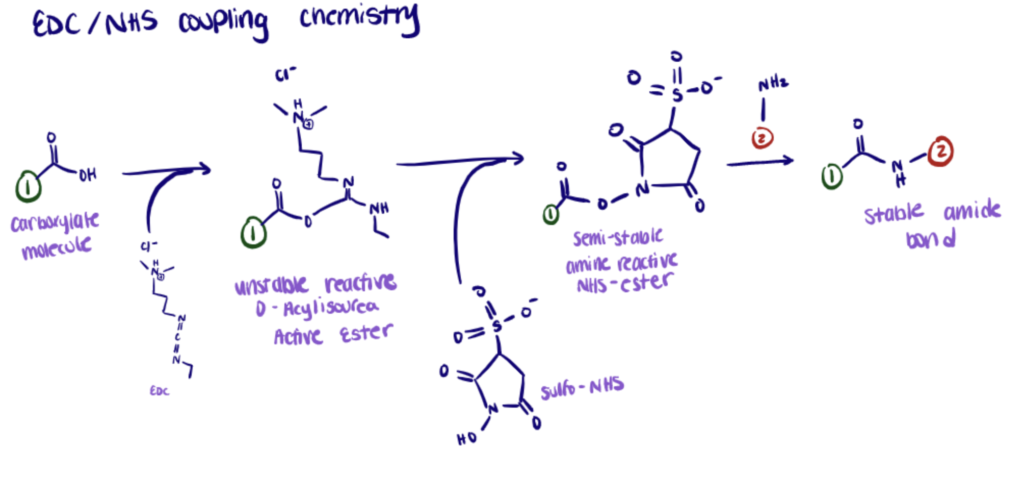
One application of EDC/NHS coupling chemistry is explained in our article about nanobody bioconjugation.
EDC Coupling Protocol
EDC coupling involves the activation of carboxyl groups and subsequent nucleophilic attack by primary amines. Thiol containing compounds can be added to quench the reaction mixture.
As a functional component of bifunctional crosslinker conjugation, EDC reacts and activates the carboxyl groups which result in an amine-reactive O-acylisourea intermediate. This intermediate reacts spontaneously with an amine to crosslink two molecules through a stable amide bond.
For this reaction, it is crucial to consider that the amine-reactive intermediate (O-acylisourea) is unstable in aqueous solutions; thus, it is necessary to quench the EDC activation reaction with a thiol-containing compound.
Despite being described as a 2-step coupling protocol where step 1 includes the activation of carboxyl groups and step 2 involves the subsequent reaction with an amine, this protocol is better explained when divided into the five steps described below (G-Biosciences, 2017).
Step 1. Equilibrate Reagents To Room Temperature
To avoid poor crosslinking, this step is essential due to the hygroscopic characteristics of the reagents.
Step 2. Add Reagents To Reaction Mixture
Dry EDC, alongside NHS or sulfo-NHS if used to stabilize O-acylisourea, are added to the amine-containing solution in the conjugation buffer for 15 minutes at room temperature.
Step 3. Quench EDC Coupling Reaction
A thiol-containing compound such as 2-mercaptoethanol is added.
Step 4. Add The Amine-Containing Compound To Crosslink
The compound is added in an equimolar amount and the reaction takes place at room temperature for two hours.
Step 5. Quench The Reaction And Purify Coupled Product
The reaction is quenched with hydroxylamine. For more information, consult the complete protocol here.
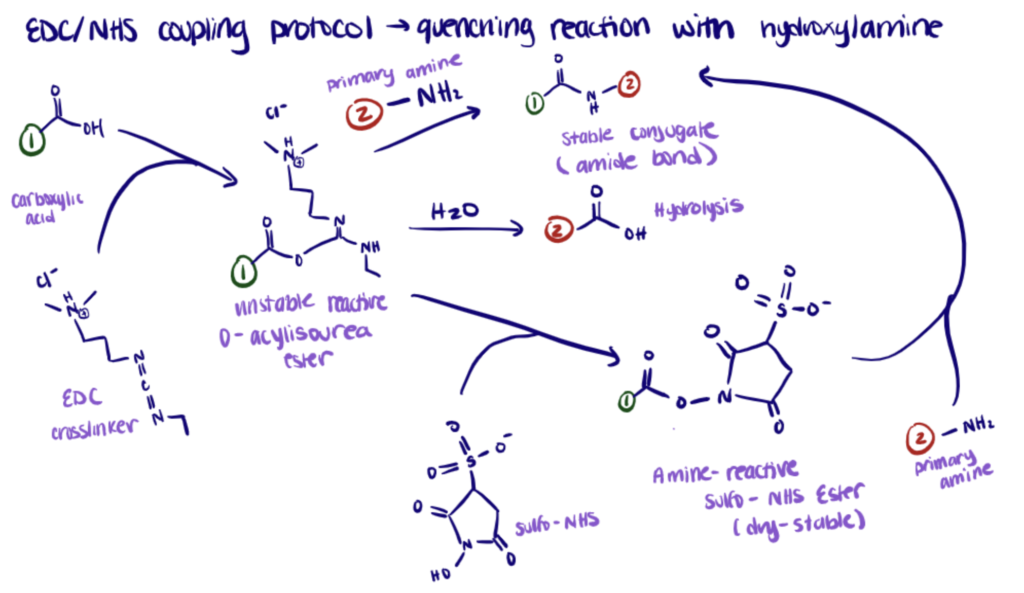
Applications Of EDC Chemistry In Literature
Applications of EDC chemistry include the synthesis of anticancer cyclic heptapeptides, stabilization of cellulose nanofibrils, and bioconjugation of hapten carrier proteins.
Application 1. Synthesis Of Cyclic Heptapeptide With Anticancer Activity
For this application, researchers synthesized a cyclic heptapeptide named Euryjanicin A, originally isolated from the marine sponge Prosuberites laughlini (Anand et al., 2019). The synthesis proceeded via a solution-phase peptide synthesis technique using EDC as a coupling reagent and triethylamine (Et3N) as a base.
The importance of the synthesized cyclic heptapeptide lies in its high cytotoxic activity against HeLa cell lines concerning standard drug cisplatin.
The synthetic route took place with a disconnection strategy. Overall, the cyclic heptapeptide molecule was detached into three dipeptide units (1, 2, and 3) and a single amino acid unit (4). As the image below shows, they were coupled, obtaining a linear fragment that was then cyclized into the cyclic heptapeptide (8).
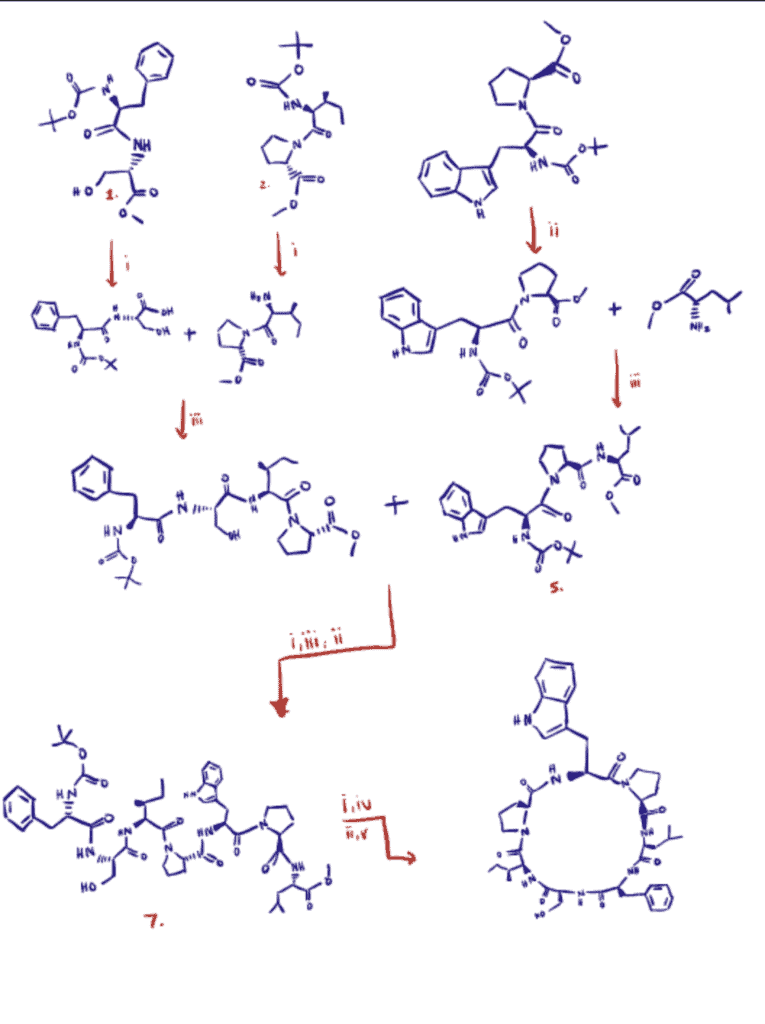
Application 2. Cellulose Nanofibrils (CNF) Stabilized With Amine-Terminated Polyethylene Glycol (PEG)
Here, researchers sought to covalently modify the cellulose nano fibrillar surface with a polymer to improve steric stabilization (Kaldéus et al., 2018). EDC/NHS coupling was used to form amide bonds between the carboxylate groups on CNF and amine-functional PEGs. In other words, the method was used to covalently graft methoxy polyethylene glycol amine (mPEGamine) chains onto the nanocellulose surface. It was also essential for them to accomplish a stable colloidal nano fibrillar system with as low amount of stabilizing polymer as possible.
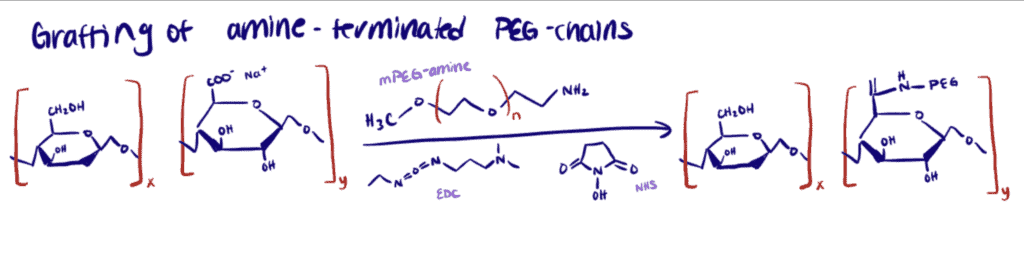
Application 3. Hapten Carrier Conjugation For Immunogenicity
Haptens are small chemical molecules that do not produce immune reactions. Once they are attached to macromolecules called carriers, they can become immunogenic (Lemus and Karol, 2008). Antibody conjugation methods are available to couple haptens to carrier proteins. Still, the choice of the appropriate conjugation chemistry depends on the functional groups available on the hapten and the effect of conjugation on biological and antigenic properties mainly.
Considering that most proteins contain exposed lysine’s and carboxyl groups, EDC bioconjugation is commonly used for hapten-carrier conjugation (ThermoFisher Scientific).
Also, EDC-mediated conjugations are expected to result in polymerization due to both peptides and antigens containing both primary amines and carboxylates. As a consequence, some peptides will conjugate to themselves as well as to the carrier protein.
Such polymerization is not always a disadvantage for immunogenicity and antibody production. Some polymerized peptides on the surface of the carrier may enhance the immunogenicity of the peptide, producing a more significant antibody response.
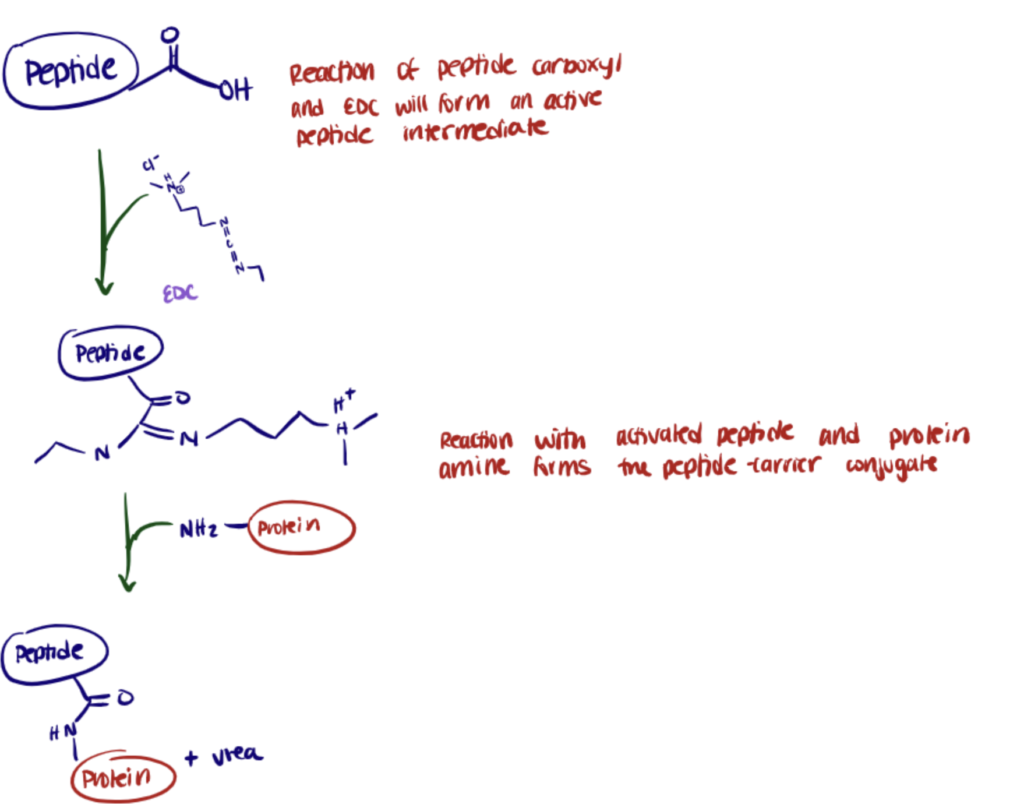